An fMRI investigation of the neural correlates underlying the autonomous sensory meridian response (ASMR)
Bioimpacts, 8(4), 295-304; DOI:10.15171/bi.2018.32
Original Research
An fMRI investigation of the neural correlates underlying the autonomous sensory meridian response (ASMR)
Bryson C. Lochte1, Sean A Guillory1, Craig A. H. Richard2,*, William M. Kelley1
1
Center for Cognitive Neuroscience, Department of Psychological and Brain Sciences, Dartmouth College, Hanover, NH, USA
2
Department of Biopharmaceutical Sciences, Bernard J. Dunn School of Pharmacy, Shenandoah University, Winchester, VA, USA
*Corresponding author: Craig Richard, Email: crichard@su.edu
Abstract
Introduction
: The "autonomous sensory
meridian response" (ASMR) is a neologism
used to describe an internal sensation of
deep relaxation and pleasant head tingling
which is often stimulated by gentle sounds,
light touch, and personal attention.
Methods
: An fMRI-based methodology was
employed to examine the brain activation of
subjects prescreened for ASMR-receptivity
(n=10) as they watched ASMR videos and
identified specific moments of relaxation
and tingling.
Results
: Subjects who experienced ASMR showed significant activation in regions associated
with both reward (NAcc) and emotional arousal (dACC and Insula/IFG). Brain activation during
ASMR showed similarities to patterns previously observed in musical frisson as well as affiliative
behaviors.
Conclusion
: This is the first study to measure the activation of various brain regions during ASMR
and these results may help to reveal the mechanistic underpinnings of this sensation.
Keywords: Autonomous sensory meridian response, ASMR, fMRI, Frisson, Whole brain imaging, ROI analysis
Introduction
Autonomous Sensory Meridian Response (ASMR) is a term that was coined in 2010 to describe deep feelings of relaxation often accompanied by a pleasant tingling in the scalp.1 The terms, “brain tingles” and “brain orgasm”, are also commonly used to describe these sensations.2,3 ASMR is often stimulated by gentle sounds, light touch, and personal attention from someone with a caring disposition.1,4 The personal attention could be from someone in real life, or from someone in an intentionally created video, referred to as an ASMR video.
The first peer-reviewed research study about ASMR was published in 2015 and reported that viewers of ASMR videos felt the strongest tingles in response to whispering and personal attention.5 The participants in the study reported that they watched the videos to help them to relax, fall sleep, and deal with stress. Another study published in 2017 reported similar findings.6 The study reported that ASMR videos induced the strongest ASMR via whispering, spa role-plays, hair brushing, and doctor role plays, and the reason for watching the videos was to experience ASMR, relax, fall asleep, and reduce anxiety.
ASMR has been compared to musical frisson.7 Musical frisson is also known as music chills because it usually involves a chills-down-the-spine sensation felt during peak moments of emotion in the music.8 The head tingles of the ASMR and the spine chills of musical frisson are generally described as pleasant sensations, occur while engaged in something enjoyable, and occur for a relatively short duration. A neuroimaging study of ASMR could help determine further similarities or differences between ASMR and frisson.
A recent functional magnetic resonance imaging (fMRI) study investigated the differences in the functional connectivity of the default mode network (DMN) between ASMR-sensitive and ASMR-insensitive individuals during resting state scans.9 The DMN of ASMR-sensitive individuals demonstrated regions of decreased connectivity in the precuneus and thalamus, and regions of increased and decreased connectivity in the frontal gyrus and temporal gyrus. This study showed differences between the brains of those who do and do not experience ASMR, however, no study has observed the brain activity involved in the ASMR experience itself.
Neuroimaging studies have identified the brain regions and neural mechanisms active during musical frisson. A study done with positron emission tomography (PET) demonstrated that musical frisson increases activity in the nucleus accumbens (NAcc), insula, and the supplementary motor areas (SMAs), but decreases activity in the medial prefrontal cortex (mPFC).10 Another study, which included PET and fMRI imaging, showed that frisson was associated with dopamine release in the NAcc.11
A priori brain regions associated with musical frisson and other potentially related processes such as self-relevant thought, empathy, mirror neuron activation, and somatosensory activation, were used to analyze the fMRI data. ASMR-sensitive individuals were recruited, underwent scanning while watching ASMR videos, and identified specific moments of relaxation and tingles during the scanning procedure. Brain regions of interest (ROIs) were analyzed for increased activity during relaxation without tingles, relaxation with tingles, and moments of simulated touch in the videos. This is the first study to measure the activation of various brain regions during ASMR.
Materials and Methods
Study approval and procedures
The study was approved by the Committee for the Protection of Human Subjects at Dartmouth College. Committee approval was acquired prior to initiation of the study, written informed consent was obtained from all participants, and all data were collected at Dartmouth College in Hanover, New Hampshire. All participants were screened for fMRI-compatibility (e.g., the absence of pacemakers or metal implants) and right-handedness.
Participants
ASMR-sensitive individuals were recruited via an advertisement posted to various online ASMR forums. The advertisement asked for ASMR-sensitive volunteers living in the northeastern United States who would be willing to travel to Dartmouth College in Hanover, New Hampshire. The advertisement also stated that the study would involve watching ASMR videos while undergoing a fMRI scan. Individuals were compensated for time and travel, but this compensation was not mentioned prior to the study visit to discourage any incentive for falsely reporting ASMR sensitivity.
ASMR sensitivity was defined by an ability to consistently report a relaxing and pleasurable tingling sensation multiple times while watching online videos tagged as “ASMR”. The content of the selected ASMR videos included an actor interacting with an object, the viewer, or another actor with characteristic ASMR triggers such as whispering, crinkling, tapping, caring dispositions, task demonstration, personal attention, ear-to-ear vocals, hair touching, and/or role-playing. Videos with music, atypical ASMR triggers, or unusual visual elements (e.g. black and white film, video “cuts”, still picture with audio) were not included. Individuals who reported ASMR (prior to or after watching selected videos) without a tingling sensation, in response to music, or without any external stimulation were excluded from the study.
Overall, 25 individuals were initially considered based on responses to an online advertisement, 18 individuals traveled to Dartmouth College for further screening, thirteen individuals were scheduled for scanning, and eleven individuals completed the scanning procedures. Data from ten subjects were used for the analysis, after the data from one individual was removed due to excessive head motion during scanning.
Stimuli
All participants reviewed 5 different full-length ASMR videos and each participant then selected 5, seven-minute clips from the full-length videos which strongly stimulated their ASMR. Subjects were instructed to avoid watching their selected video clips for the 48 hours prior to their scan to prevent any desensitization effects. During the scan, the subjects viewed their 5 selected ASMR video clips in a row, with a 30-second break between the clips. At the end of each video clip was a 2-second alarm to interrupt any state of deep relaxation followed by a thirty-second view of a fixation cross to baseline the subject prior to viewing their next ASMR video clip (Fig. 1).

Example of a complete scan session for the participants. Each participant viewed 5 sequential 7-minute ASMR video clips with a 30 second viewing of a fixation cross after each video clip.
Procedure
Subjects were given a box displaying 3 buttons. They were instructed to press a specific button to indicate one of 3 sensations: a baseline sensation to account for brain activation associated with pressing a button, a relaxing sensation experienced as a pleasant, relaxed, or trance-like state, or a tingling sensation experienced as an acute, pleasurable tingle feeling in the back of the head or neck. The subjects were instructed to press one of the 3 buttons at all times throughout the videos.
Simulated touch moments (periods when the viewer appears to be touched by an actor in the video) were identified after the study completion and included as events in a separate analysis. Touch events were not a requirement during the ASMR video selection process for each participant, but they were identified in the ASMR videos for 8 out of the ten participants.
Data analysis
Data were analyzed for 5 regressors of interest: (1) fixation periods between videos when subjects observed a fixation cross for 30 seconds, (2) baseline periods during the videos when the subjects pressed a button to indicate they did not feel a relaxing or tingling sensation, (3) relaxing periods, without tingling, during the videos indicated by a button press from the subjects, (4) tingling periods during the videos indicated by a button press from the subjects, and (5) touch events during the videos which were determined by the investigators to be when the actor in the video pretends to touch the viewer. Moments, when the subjects forgot to press a button during a session, were noted and not analyzed as data.
Apparatus
Imaging was performed on a Phillips 3.0-T Philips Intera Achieva scanner (Philips Medical Systems, Bothell, WA, USA) equipped with a SENSEitivity Encoding head coil. While situated in the scanner bed, subjects were equipped with a display screen and stereophonic headphones so that auditory and visual stimuli could be presented. Visual stimuli were generated using SuperLab 4.0 (Cedrus Corporation, San Pedro, CA, USA), and projected with an Epson ELP-7000 LCD screen (Epson America, Inc., Long Beach, CA, USA) located at the head end of the scanner bore. Participants viewed the screen through a mirror mounted on the head coil. Cushions were used to minimize head movement.
Imaging
The scanning sequence involved one scout image, one sense reference image, one high-resolution anatomical run, and 3 functional runs. Anatomical images were acquired using T1-weighted imaging (MP-RAGE; 160 sagittal slices, echo time = 4.6 ms, repetition time = 9.9 ms, flip angle = 8°, voxel size = 1x1x1 mm). Functional images were collected using T2*-weighted echo-planar imaging sequence (echo time = 35 ms, repetition time = 2000 ms, flip angle = 90° and FOV-240 mm). Throughout 3 functional runs, 1046 sets (36 Axial slices, 4.0 mm thick with 0.5 mm skip, 3.0 × 3.0 mm in-plane resolution) were collected, allowing for whole brain coverage.
fMRI Data analyses
The fMRI data were analyzed using Statistical Parametric Mapping software (SPM8; The Wellcome Trust Centre for NeuroImaging, London, UK). Raw fMRI data were pre-processed using standard procedures to correct for motion, slice acquisition, and field inhomogeneities. Functional images were then normalized to a standard anatomical space (MNI-152). Spatial smoothing was applied to the normalized functional images using a Gaussian kernel (6 mm Full Width at Half Maximum).
Six-millimeter spherical ROIs were centered on the peak voxel of task sensitive clusters for the t-test analyses. Parameter estimates for each ROI were extracted for each participant for each category. In order to explore which categories were selective for each ROI, the estimates were submitted to offline-paired t-tests. Contrast images for each subject, comparing task effects for each condition with baseline, were then submitted to a second-level one sample t-test for baseline and paired t-test for between categories.
For each subject, a GLM incorporating task effects and covariates of no interest was convolved with a canonical hemodynamic response function. The covariates of no interest included instruction and error trials, a linear trend, and 6 motion parameters derived from realignment corrections. The GLM was used to compute parameter estimates for comparisons at each voxel. The individual contrast images underwent a random-effects analysis to create mean t-images. This analysis allowed a comparison of all trial types to baseline to identify task-related activations.
Differences between individual trials could be examined by generating contrast images. Individual contrast images were then submitted to a second-level, random effect analysis to create mean t-images. The threshold was set to P < 0.05 and corrected for false discovery rate (FDR).
Regions of Interest (ROIs)
The data were analyzed using various a priori ROIs (Table 1). Because no studies have been published on the effect of ASMR videos on brain activity, these ROIs were selected based on various theoretical mechanisms underlying ASMR.
Table 1. Regions of Interest selected for their potential involvement during ASMR
|
Neural Associations
|
Regions of Interest
|
MNI coordinates
|
Self-Relevant Thought |
|
R medial prefrontal cortex (mPFC) |
10, 52, 4 |
|
L medial prefrontal cortex (mPFC) |
-6, 54, 9 |
|
Ventral anterior cingulate cortex (vACC) |
0, 23, -9 |
Reward Activation |
|
R nucleus accumbens (NAcc) |
14, 10, -10 |
|
L nucleus accumbens (NAcc) |
-21, 9, -10 |
Emotional Arousal |
|
Dorsal anterior cingulate cortex (dACC) |
-2, 24, 38 |
|
L supplementary motor areas (SMA) |
-4, 14, 54 |
|
R supplementary motor areas (SMA) |
6, 8, 60 |
|
R insula/inferior frontal gyrus (I/IFG) |
38, 24, -2 |
|
L insula/inferior frontal gyrus (I/IFG) |
-42, 18, 0 |
Somatosensory Processing |
|
L SII: Mandibular Angle |
-62, -21, -20 |
|
L SII: Cheek |
-57, -24, 20 |
|
L SII: Lip |
-51, -24, 17 |
|
L SII: Forehead |
-59, -23, 17 |
|
L SII: Foot |
-47, -24, 20 |
Mirror Neuron Clusters |
|
R inferior parietal lobule (IPL) |
45, -26, 42 |
|
L inferior parietal lobule (IPL) |
-50, -29, 42 |
|
R posterior inferior frontal gyrus (PIFG) |
49, 15, 24 |
|
L posterior inferior frontal gyrus (PIFG) |
-53, 26, 9 |
|
Ventral premotor cortex (VPC) |
56, -16, 39 |
The behavioral effects of ASMR in humans are similar to those seen in animals during grooming. Grooming in non-human primates results in a calm, still state, followed by reduced anxiety and improved mood.12 A popular style of ASMR video involves the actor interacting with the camera as though it were the viewer, giving the video a first-person perspective. These videos, in particular, seem to create a ‘virtual grooming’ experience. The popularity of these videos could indicate the importance of self-relevant cognition in conjunction with the grooming sensation. The a priori regions selected to examine self-relevant thought included the bilateral mPFC and the ventral anterior cingulate cortex (vACC).13
Neuroimaging research has shown that frisson, in response to music, is associated with increased activity in areas of reward, motivation, emotion, and arousal.10 ASMR could have similar mechanistic underpinnings with the frisson sensation. For this reason, ROIs were selected for regions corresponding to reward activation; the nucleus accumbens (NAcc), as well as regions corresponding to emotional arousal; the dorsal anterior cingulate cortex (dACC), the left and right supplementary motor area (SMA), and the bilateral regions between the insula and inferior frontal gyrus (insula/IFG).11,14
If ASMR were a vestigial grooming response, activations in the somatosensory areas of the brain may be expected when subjects viewed ASMR videos – especially during moments of simulated physical contact. ROIs were located in the secondary somatosensory cortex on the left side of the brain, with specific locations corresponding to touch sensations in the mandibular angle, cheek, lip, forehead, and foot, with the foot serving as a control region that is not typically touched during ASMR videos.15
ASMR videos commonly include an individual carefully and repetitively performing a fine motor task. ASMR could have developed as an evolutionarily favorable trait because it encourages learning through observation. If ASMR is a response to observational learning, it should show robust activation in the mirror neuron systems, which play an important role in the perception and representation of observed movements.16 Five ROIs were selected to investigate activation in the mirror neuron system, including the right and left inferior parietal lobule (IPL), the right and left posterior inferior gyrus and the ventral premotor area, all of which have been shown in a meta-analysis as highly active mirror neuron clusters.17
Results
Behavioral results
Ten ASMR-sensitive subjects (3 female, 7 male, mean age 23.6, age range 18–33) participated in the study. Participants viewed 5 sequential ASMR videos of 7 minutes duration each, with a 30-second viewing of a fixation cross after each video (Fig. 1). Participants pressed one of 3 buttons during the videos to indicate baseline sensations, relaxing sensations, or tingling sensations. Duration, occurrences, and total time of the sensations experienced by the participants while watching the ASMR videos are shown in Fig. 2.
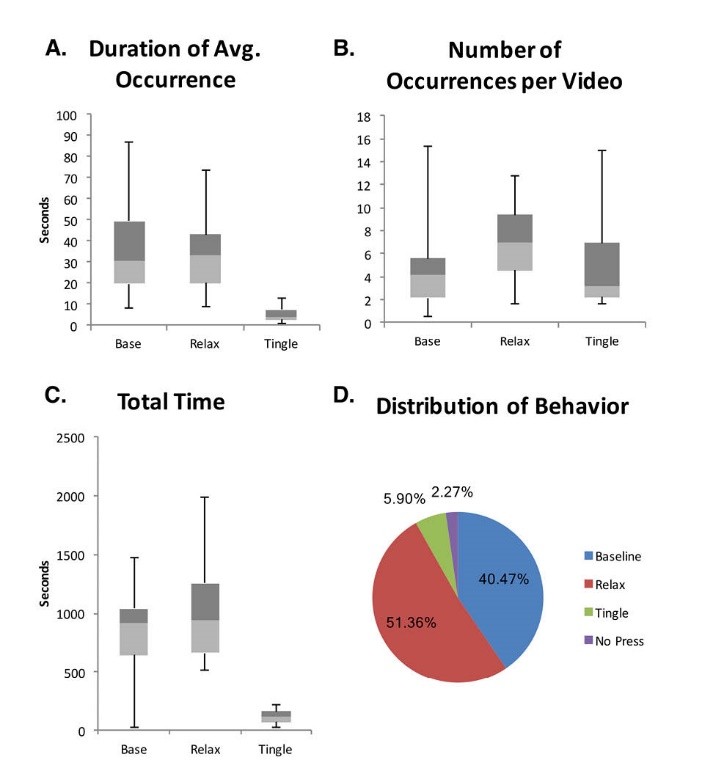
Sensations experienced by the participants while watching ASMR video clips. Participants (n = 10) pressed a button while viewing the videos to indicate timing of baseline sensations (Base), relaxing sensations (Relax), or tingling sensations (Tingle). (A) Duration of each sensation. (B) Number of occurrences for each sensation per video. (C) Total duration for each sensation. (D) Percent of each sensation for all sessions including percent of time when a button was not pressed (No Press). Boxplots show minimum values, maximum values, and median values separating the 2nd quartile values (light gray) from the 3rd quartile values (dark gray).
Fig. 2A shows the time duration of the different sensations for each subject. Baseline sensations occurred for an average of 40.2 seconds (median: 30.8, SD: 26.7, range: 8.4-86.7 seconds), relaxation without tingles occurred for an average of 37.5 seconds (median: 33.6, SD: 22.0, range: 8.9–74.0 seconds), and tingles occurred for an average of 6.3 seconds (median: 4.0, SD: 4.4, range: 1.3–12.9 seconds).
Fig. 2B shows the number of occurrences for each sensation per video. Baseline sensations occurred an average of 5.06 times (median: 4.1, SD: 4.3, range: 0.6 – 15.4 times), relaxation without tingles occurred an average of 7.22 times (median: 7.0, SD: 3.5, range: 1.6–12.8 times), and tingles occurred an average of 5.62 times (median: 3.2, SD: 4.4, range: 1.6–15.0 times).
Fig. 2C shows the average total time of each sensation per participant session. Baseline sensations occurred for an average total time of 849.8 seconds (median: 912.3, SD: 475.2, range: 25.2-1473.6 seconds), relaxation without tingles occurred for an average total time of 1078.6 seconds (median: 937.4, SD: 510.6, range: 511.5–1981.9 seconds), and tingles occurred for an average total time of 123.8 seconds (median: 119.3, SD: 63.1, range: 28.0–215.7 seconds).
The percent of the total ASMR video viewing time spent by all participants pressing a button for each sensation is shown in Fig. 2D. The participants reported baseline sensations for 40.5% of the time, relaxation without tingles for 51.4% of the time, tingles for 5.9% of the time, and the participants forgot to press a button for 2.3% of the total ASMR video viewing time.
Whole brain activations
Whole brain activations were observed during the video clips and compared to the 30 second fixation period between the videos. As expected, the scans from various moments during the video clips showed increased activation in auditory and visual cortices (data not shown). Whole brain activations during the videos were additionally observed and compared for the specific moments of the baseline sensations, relaxation sensations, and tingling sensations (Figs. 3 and 4).
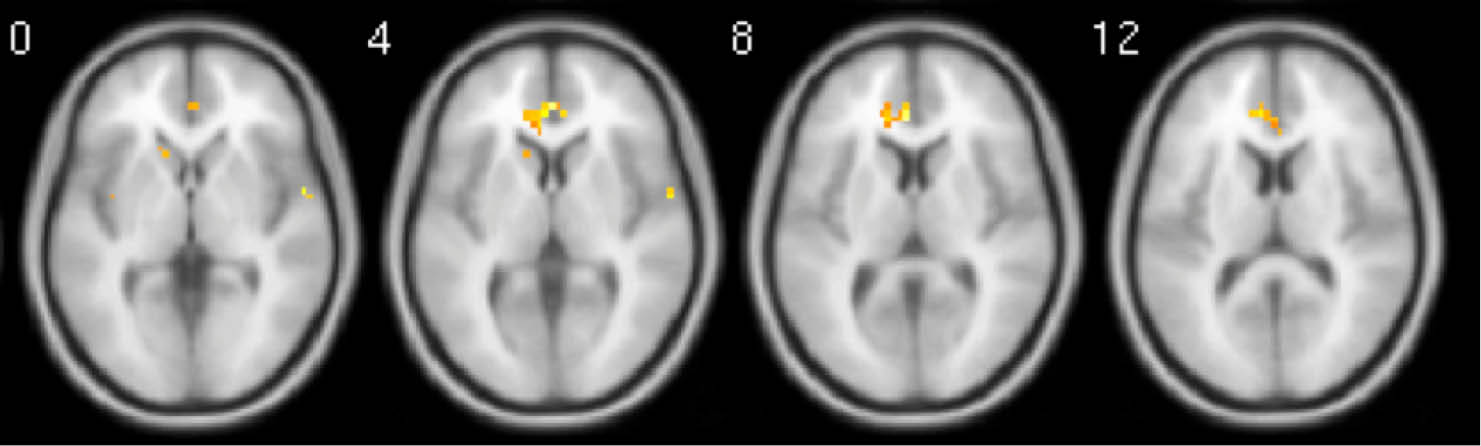
Whole brain activation during relaxing moments when contrasted with baseline moments. Activations have been FDR corrected and were only observed in the mPFC. Images are average brain activations across all subjects.

Whole brain activation during tingling moments when contrasted with baseline moments. Activations have been FDR corrected and were observed in the mPFC, as well as, a variety of regions notably surrounding the NAcc and Insula. Images are average brain activations across all subjects.
When relaxing moments were compared with baseline moments, a small but significant cluster of activation appeared in the mPFC after FDR correction, but not for the vACC (Fig. 3). Other horizontal slices showed little significant activity. When tingling moments were compared with baseline moments, multiple and significant clusters of activation appeared in the mPFC, insula, and NAcc after FDR correction (Fig. 4).
ROI activations
Specific ROI were analyzed for activation based on their potential relevance to ASMR (Table 1). Each ROI was analyzed during the periods of baseline sensations, relaxing sensations, tingle sensations, and touch events and reported in values relative to the 30 second fixation period (Figs. 5, 6, 7).
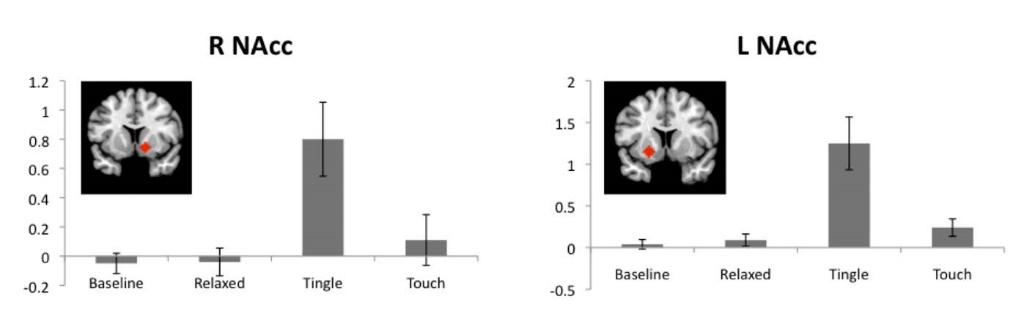
ROIs associated with reward pathways. NAcc activations for baseline, relaxing, tingle, and touch periods relative to a fixation cross period. Red diamonds indicate the location of each ROI. R: right, L: left, NAcc: nucleus accumbens. Values are means with standard errors.
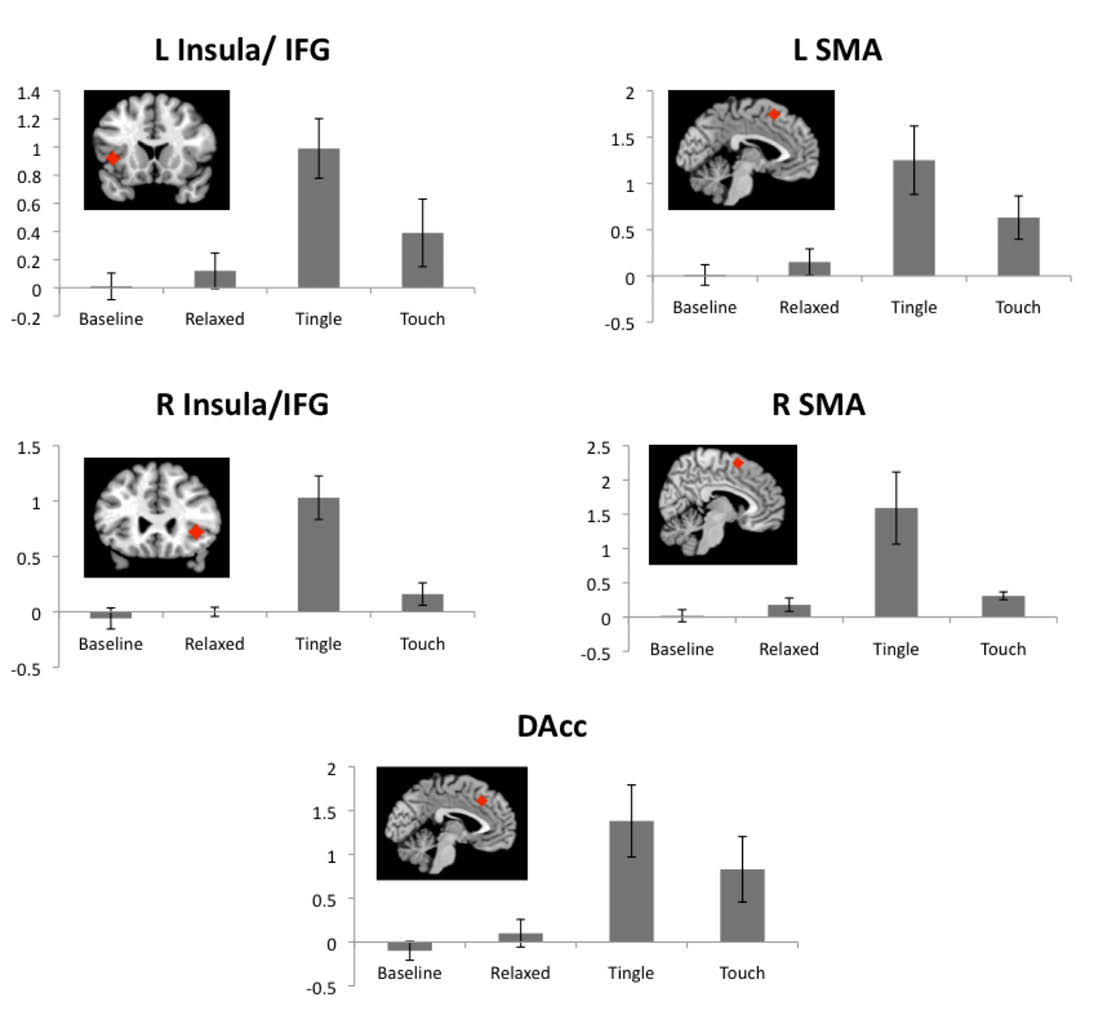
ROIs associated with emotional arousal and empathy. Insula/IFG, SMA, and DAcc activations for baseline, relaxing, tingling, and touch periods relative to a fixation cross period. Red diamonds indicate the locations of each ROI. R: right, L: left, IFG: inferior frontal gyrus, SMA: supplementary motor areas, DAcc: dorsal anterior cingulate cortex. Values are means with standard errors.
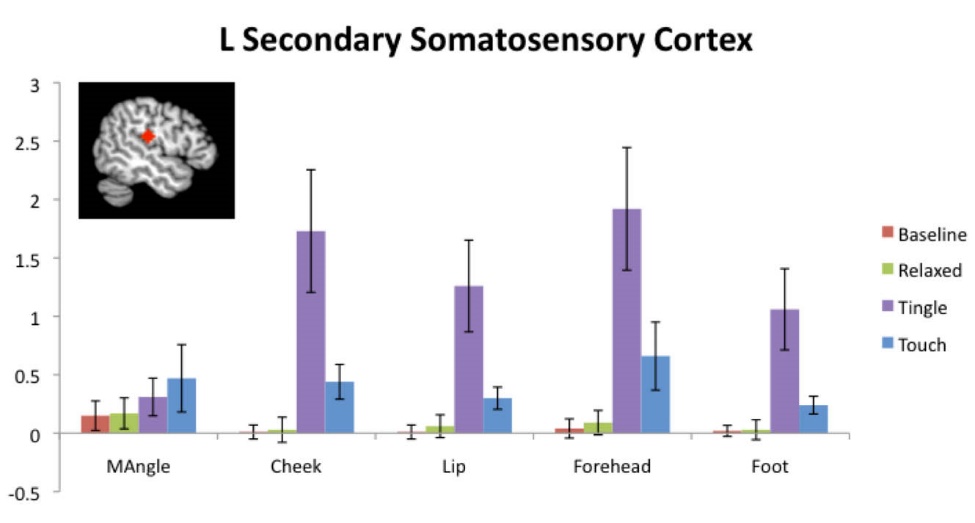
ROIs associated with the perception of touch. Left secondary somatosensory cortex activations in the mandibular angle, cheek, lip, forehead, and foot regions for baseline, relaxing, tingling, and touch periods relative to a fixation cross period. Red diamond is specific to the ROI for lip. Values are means +/- standard errors of the mean.
Fig. 5 shows the ROI results associated with reward pathways. The right NAcc (t = 3.80, P = 0.004) and left NAcc (t = 3.53, P = 0.006) demonstrated highly significant positive activation during tingle sensations but not during baseline or relaxing sensations. Touch events also showed slightly significant activation in the left NAcc (t = 2.31, P = 0.046) but not in the right NAcc.
Figure 6 shows the ROI results associated with emotional arousal and empathy. The tingling sensation was highly significant for all emotional arousal regions of interest, including the dACC (t = 3.39, P = 0.008), left SMA (t = 3.12, P = 0.012), right SMA (t = 2.96, P = 0.016), left insula/IFG (t = 4.76, P = 0.001), and right insula/IFG (t = 6.44, P < 0.001). Although these regions also demonstrated increased activation during touch events, only the left SMA (t = 2.69, P = 0.025) and right SMA (t = 5.54, P < 0.001) were significantly activated. Significant activations of these regions were not observed during baseline or relaxation sensations.
Fig. 7 shows the ROI results associated with the perception of touch. The perception of touch occurred when the actor in the video pretended to touch the viewer, which occurred for an average total time of 174 seconds per participant (data not shown). The tingling sensation demonstrated highly significant activity in the somatosensory regions corresponding to the cheek (t = 3.30, P = 0.009), lip (t = 3.20, P = 0.011), forehead (t = 3.66, P = 0.005) and foot (t = 3.03, P = 0.014). Touch events also showed significant positive activation in the somatosensory regions corresponding to the cheek (t = 2.96, P = 0.016), lip (t = 3.09, P = 0.013 ), forehead (t = 2.27, P = 0.049) and foot (t = 3.22, P = 0.011).
ROI results associated with action observation and mirror neuron clusters showed less consistent results (data not shown). Tingling sensations were associated with significant activation of the left IPL (t = 3.16, P = 0.012) and VPC (t = 2.44, P = 0.037), but not with significant activation in the right IPL, left PIFG, or right PIFG. No significant activations were observed in these regions for baseline sensations, relaxation sensations, or touch events.
Discussion
This is the first study to measure the activation of various brain regions during ASMR. Participants with ASMR-sensitivity observed ASMR videos during fMRI scanning and indicated specific moments of pleasurable head tingling, relaxation without head tingling, or neither. One of the general, yet important, findings of this study is that ASMR can occur during a fMRI procedure. Quiet and relaxed conditions have been reported to be important for experiencing ASMR.5 fMRI scanners are large and loud machines which involve the patient lying inside them while the scanning occurs. This is the first research study to report the occurrence of ASMR during fMRI scanning which should encourage additional neuroimaging studies on ASMR.
We observed significant brain activation in the mPFC during ASMR. This brain region is associated with self-awareness, social cognition, and social behaviors including grooming.13,18,19 Although the participants were watching recorded videos, these results may indicate that ASMR videos activate the brain similarly to actual social engagement. Oxytocin has been shown to bind to receptors in the mPFC and mediate relaxation responses.20 The activation of the mPFC during ASMR may also suggest a potential contribution of oxytocin to the relaxing sensations during tingles.
The network of the dACC, SMA, and insula are also considered to be involved in empathy.14 The activation of these regions during ASMR tingles provides support for the association of ASMR with social cognition and caring feelings towards others. A 2017 study reported that ASMR-sensitive individuals scored higher for empathic concern than individuals who do not experience ASMR.6 The association of ASMR and increased empathy may suggest that ASMR could also be associated with personality traits and mental health. Two prior studies utilized the Big Five Inventory to assess 5 personality traits in ASMR-responders and non-responders.6,21 Both studies showed that ASMR-responders had significantly higher scores for the trait of ‘openness-to-experience’ than non-responders. A future study could utilize the Temperament and Character Inventory, which includes empathy and other subscales within 7 dimensions of personality traits, to further assess relationships between ASMR, personality development, personality traits, social cognition and mental health.22
Several somatosensory regions involved in touch perception were measured to further investigate a potential association between ASMR and a vestigial grooming pathway. Significant brain activation during brain tingles was observed in the regions which were being virtually touched by the actor in the video. However, there was additional activation in the foot region which was not being virtually touched. This may indicate that the coordinates are not accurate enough to represent simulated touch events in ASMR videos.
During the tingling events, there was inconsistent activation in the ROIs identified for the mirror neuron system. Furthermore, whole-brain neural activation during tingling sensations seems to show comparatively less activation in mirror neuron regions. Therefore, this study does not provide strong support that watching ASMR videos and experiencing tingles stimulates mirror neuron activation.
The tingles of ASMR have some similarities to the chills of frisson, which are typically induced by music. Music chills have been strongly associated with activation of the NAcc, dACC, SMA, and insula.20,23 We demonstrated that these same regions were also strongly activated during ASMR tingles. Many prior studies of the NAcc have elucidated this area to be a key brain region for reward, satisfaction, and emotion – often involving dopamine.24 Dopamine has been shown to be released in the NAcc during music chills and may therefore also be released in the NAcc during ASMR tingles.11 The similar pattern of activation of both ASMR and frisson could explain their subjective similarities, such as their short duration and tingling sensation within a related neuromechanistic model. Nevertheless, individuals who have experienced both, report that ASMR and frisson are qualitatively distinct and the types of stimuli that trigger each respective sensation are completely different.7 The perceived differences between ASMR and frisson could involve the mPFC, this brain region demonstrated increased activation during tingling in our study but was reported to have decreased activation during frisson.10 Further studies can help to identify the shared similarities and unique differences between these 2 pleasant sensations.
The broadest explanation of our results and for most ASMR triggers is that they are related to affiliative behaviors. Affiliative behaviors are the caring behaviors which occur between parents and children, romantic partners, and friends.25 These behaviors involve close interpersonal bonds and can include grooming behaviors along with a wider array of care-giving and care-receiving behaviors. The attention-receiving or observation-granting behaviors in many ASMR videos have similarities to being cared for or to looking after someone. Studies utilizing fMRI have shown some affiliate behaviors involve strong activation of the mPFC, NAcc, insula, and IFG – all regions strongly activated in our study.25 The neurohormones widely reported to be responsible for most affiliative behaviors include dopamine, oxytocin, and endorphins.25,26 These neurohormones are known for inducing feelings of comfort, relaxation, and sleepiness – feelings often reported with ASMR.5,6
The benefits of healthy relationships and affiliative behaviors have been well documented and specifically attributed to the neurochemicals released during interpersonal bonding.26 These positive social interactions occur during infancy, youth, adulthood, and elderhood and have been shown to increase oxytocin, decrease stress hormones, and decrease anxiety-like responses and behaviors.26ASMR may be activating the brain regions and releasing neurochemicals normally associated with affiliative behaviors. This may help to explain why ASMR is reported to help individuals to relax, deal with stress, decrease their anxiety, and fall asleep more easily.5,6
Specifically, Barratt et al reported that 98% of participants watch ASMR videos to relax, 82% watch the videos to help them sleep, 70% watch the videos to deal with stress, and 80% reported a positive effect on their mood.5 Janik McErlean et al reported that 86% of participants watch ASMR videos to relax or trigger ASMR, 41% watch videos to help them sleep, and 11% watch the videos to reduce their anxiety.6 These published results, along with the thousands of similarly-themed anecdotes posted to ASMR videos, forums, Facebook groups, and websites, highlight a potential clinical application of ASMR.27
ASMR is already being unintentionally stimulated in clinical settings due to the personal attention, light touch, gentle vocals, and caring behaviors of clinicians.28 The potential clinical benefits of purposely stimulating ASMR as part of a treatment plan are not currently known. Recorded ASMR triggers (e.g., video and audio files) or in-person ASMR triggers (e.g., light touch and personal attention) could be tested as a co-treatment or as an alternative treatment to current evidence-based therapies. Initial clinical trials could focus on patients with mild or treatment-resistant forms of anxiety or insomnia who would be pre-screened for ASMR sensitivity. A better physiological understanding of ASMR may be required before initiating clinical trials. Basic physiological studies should be done in healthy individuals to establish the effects of ASMR triggers on heart rate, respiratory rate, blood pressure, cortisol levels, galvanic skin response, EEG activity, and other indicators of physical and mental state. These future investigations of ASMR could help to elucidate its potential applications in the management of conditions like anxiety disorders, insomnia, and depression.
There were several limitations of our fMRI study. Some of our results, which lacked statistical significance, could have been due to the small sample size. Although our participants experienced ASMR during MRI scanning, it is still possible that some of their responses were reduced due to the scanning experience. Our study also lacked a comparison group. It would be interesting to compare fMRI results between a group of ASMR sensitive individuals and a group of individuals who do not experience ASMR. Additional control stimuli, like music and other relaxing video types, could also help to elucidate the unique or non-unique nature of ASMR. Assessing ASMR with quantitative electroencephalography in conjunction with low-resolution electromagnetic tomography, another method often used to assess neural mechanisms associated with a specific stimulus, could help to provide further insights or confirmation of involved brain regions.29 Overall, our investigation should be interpreted as a pilot study for more comprehensive fMRI studies and other explorations into ASMR.
Conclusion
Our fMRI results demonstrated that there was significantly increased activation in specific areas of the brain during moments of ASMR “tingling” compared to non-tingling moments. The brain regions found most active during the tingling sensations were the nucleus accumbens, mPFC, insula and secondary somatosensory cortex. These results are the first to demonstrate unique neural activation associated with the experience of ASMR. Overall, these results suggest that the ASMR videos are generating tingling and relaxing sensations by activation of brain regions previously observed during experiences like social bonding and musical frisson.
Acknowledgments
This work was supported by the Dartmouth College Office of Undergraduate Research, Hanover, NH.
Funding sources
Funding was provided by a Senior Honors Thesis Grant through the Dartmouth College Office of Undergraduate Research, Hanover, NH.
Ethical statement
The study was approved by the Committee for the Protection of Human Subjects at Dartmouth College.
Competing interests
The authors declare no conflict of interests.
Authors contribution
BCL: conceptualization, data handling, experiments design, data analysis, data presentation, draft preparation, study consultation, writing and reviewing. SAG: data handling, data analysis, data presentation, study validation, provision of study materials and equipment. CAHR: data interpretation, draft preparation, writing and reviewing. WMK: provision of study materials and equipment, supervision, project administration.
References
- Copeland L. How Researchers Are Beginning to Gently Probe the Science Behind ASMR. Smithsonian Magazine. 2017; https://www.smithsonianmag.com/science-nature/researchers-begin-gently-probe-science-behind-asmr-180962550/.
- Etchells P. ASMR and 'head orgasms': what's the science behind it? The Guardian. 2016; https://www.theguardian.com/science/head-quarters/2016/jan/08/asmr-and-head-orgasms-whats-the-science-behind-it.
- Marks A. The Tingle Makers, Open for Business. New York Times. 2018; https://www.nytimes.com/2018/02/15/nyregion/tingling-sensation-of-asmr-stimulation.html.
- Sackett D. Why Is My Brain Tingling? Scientific American. 2017; https://blogs.scientificamerican.com/observations/why-is-my-brain-tingling.
- Barratt EL, Davis NJ. Autonomous Sensory Meridian Response (ASMR): a flow-like mental state. PeerJ. 2015; 3: e851. doi: 10.7717/peerj.851. [Crossref]
- Janik McErlean A, Banissy M. Assessing individual variation in personality and empathy traits in self-Reported Autonomous Sensory Meridian Response. Multisens Res. 2017; 30(6): 601-13 doi: 10.1163/22134808-00002571. [Crossref]
- del Campo MA, Kehle TJ. Autonomous sensory meridian response (ASMR) and frisson: Mindfully induced sensory phenomena that promote happiness. Int J Sch Educ Psychol. 2016; 4(2): 99–105. doi.org/10.1080/21683603.2016.1130582. [Crossref]
- Goldstein A. Thrills in response to music and other stimuli. Physiol Psychol. 1980; 8(1): 126–9. doi.org/10.3758/BF03326460. [Crossref]
- Smith SD, Katherine Fredborg B, Kornelsen J. An examination of the default mode network in individuals with autonomous sensory meridian response (ASMR). Soc Neurosci. 2017; 12(4): 361-365. doi: 10.1080/17470919.2016.1188851. [Crossref]
- Blood AJ, Zatorre RJ. Intensely pleasurable responses to music correlate with activity in brain regions implicated in reward and emotion. Proc Natl Acad Sci U S A. 2001; 98(20): 11818-23. doi.org/10.1073/pnas.191355898. [Crossref]
- Salimpoor VN, Benovoy M, Larcher K, Dagher A, Zatorre RJ. Anatomically distinct dopamine release during anticipation and experience of peak emotion to music. Nat Neurosci. 2011; 14(2): 257-62. doi: 10.1038/nn.2726. [Crossref]
- Doyle GA. Behavior of Prosimians. In: Schrier AM, Stollnitz F, eds. Behavior of Nonhuman Primates. Volume 5. New York: Academic Press; 1974. p. 155–353.
- Moran JM, Macrae CN, Heatherton TF, Wyland CL, Kelley WM. Neuroanatomical evidence for distinct cognitive and affective components of self. J Cogn Neurosci. 2006; 18(9): 1586-94. doi.org/10.1162/jocn.2006.18.9.1586. [Crossref]
- Fan Y, Duncan NW, de Greck M, Northoff G. Is there a core neural network in empathy? An fMRI based quantitative meta-analysis. Neurosci Biobehav Rev. 2011; 35(3): 903-11. doi: 10.1016/j.neubiorev.2010.10.009. [Crossref]
- Nguyen BT, Inui K, Hoshiyama M, Nakata H, Kakigi R. Face representation in the human secondary somatosensory cortex. Clin Neurophysiol. 2005; 116(6): 1247-53. doi.org/10.1016/j.clinph.2005.01.018. [Crossref]
- Calvo-Merino B, Glaser DE, Grèzes J, Passingham RE, Haggard P. Action observation and acquired motor skills: an FMRI study with expert dancers. Cereb Cortex. 2005; 15(8): 1243-9. doi.org/10.1093/cercor/bhi007. [Crossref]
- Molenberghs P, Cunnington R, Mattingley JB. Brain regions with mirror properties: a meta-analysis of 125 human fMRI studies. Neurosci Biobehav Rev. 2012; 36(1): 341-9. doi: 10.1016/j.neubiorev.2011.07.004. [Crossref]
- Grossmann T. The role of medial prefrontal cortex in early social cognition. Front Hum Neurosci. 2013; 7: 340. doi: 10.3389/fnhum.2013.00340. [Crossref]
- Li CR, Huang GB, Sui ZY, Han EH, Chung YC. Effects of 6-hydroxydopamine lesioning of the medial prefrontal cortex on social interactions in adolescent and adult rats. Brain Res. 2010; 1346: 183-9. doi: 10.1016/j.brainres.2010.05.064. [Crossref]
- Sabihi S, Dong SM, Durosko NE, Leuner B. Oxytocin in the medial prefrontal cortex regulates maternal care, maternal aggression and anxiety during the postpartum period. Front Behav Neurosci. 2014; 8: 258. doi:10.3389/fnbeh.2014.00258. [Crossref]
- Fredborg B, Clark J, Smith SD. An Examination of Personality Traits Associated with Autonomous Sensory Meridian Response (ASMR). Front Psychol. 2017; 8: 247. doi: 10.3389/fpsyg.2017.00247. [Crossref]
- Cloninger CR, Svrakic DM, Przybeck TR. A psychobiological model of temperament and character. Arch Gen Psychiatry. 1993; 50(12): 975-90. doi: 10.1001/archpsyc.1993.01820240059008. [Crossref]
- Harrison L, Loui P. Thrills, chills, frissons, and skin orgasms: toward an integrative model of transcendent psychophysiological experiences in music. Front Psychol. 2014; 5: 790. doi: 10.3389/fpsyg.2014.00790. [Crossref]
- Salgado S, Kaplitt MG. The Nucleus Accumbens: A Comprehensive Review. Stereotact Funct Neurosurg. 2015; 93(2): 75-93. doi: 10.1159/000368279. [Crossref]
- Feldman R. Oxytocin and social affiliation in humans. Horm Behav. 2012; 61(3): 380-91. doi: 10.1016/j.yhbeh.2012.01.008. [Crossref]
- Colonnello V, Petrocchi N, Farinelli M, Ottaviani C. Positive Social Interactions in a Lifespan Perspective with a Focus on Opioidergic and Oxytocinergic Systems: Implications for Neuroprotection. Curr Neuropharmacol. 2017; 15(4): 543-561. doi: 10.2174/1570159X14666160816120209. [Crossref]
- Lloyd J, Ashdown T, Jawad L. Autonomous sensory meridian response: What is it? and Why should we care? Indian J Psychol Med. 2017; 39(2) doi: 10.4103/0253-7176.203116. [Crossref]
- Ahuja NK. "It Feels Good to Be Measured": Clinical Role-Play, Walker Percy, and the Tingles. Perspect Biol Med. 2013; 56(3): 442-51 doi: 10.1353/pbm.2013.0022. [Crossref]
- Cannon RL, Baldwin DR, Shaw TL, Diloreto DJ, Phillips SM, Scruggs AM, Riehl TC. Reliability of quantitative EEG (qEEG) measures and LORETA current source density at 30 days. Neurosci Lett. 2012; 518(1): 27-31. doi: 10.1016/j.neulet.2012.04.035. [Crossref]