Placenta mesenchymal stem cells differentiation toward neuronal-like cells on nanofibrous scaffold
Bioimpacts, 10(2), 117-122; DOI:10.34172/bi.2020.14
Original Research
Placenta mesenchymal stem cells differentiation toward neuronal-like cells on nanofibrous scaffold
Fatemeh Rahimi-Sherbaf1, Samad Nadri2,3, Ali Rahmani2, Atousa Dabiri Oskoei4*
1
Department of Obstetrics and Gynecology, School of Medicine, Yas Hospital, Tehran University of Medical Sciences, Tehran, Iran
2
Department of Medical Nanotechnology, School of Medicine, Zanjan University of Medical Sciences, Zanjan, Iran
3
Zanjan Metabolic Diseases Research Center, Zanjan University of Medical Sciences, Zanjan, Iran
4
Department of Obstetrics and Gynecology, Mousavi Hospital, Zanjan University of Medical Sciences, Zanjan, Iran
*Corresponding author: Atousa Dabiri Oskoei, Email: dabiri@zums.ac.ir
Abstract
Introduction:
Transplantation of stem cells with a nanofibrous scaffold is a promising approach for spinal cord injury therapy. The aim of this work was to differentiate neural-like cells from placenta-derived mesenchymal stem cells (PDMSCs) using suitable induction reagents in three (3D) and two dimensional (2D) culture systems.
Methods: After isolation and characterization of PDMSCs, the cells were cultivated on poly-L-lactide acid (PLLA)/poly caprolactone (PCL) nanofibrous scaffold and treated with a neuronal medium for 7 days. Electron microscopy, qPCR, and immunostaining were used to examine the differentiation of PDMSCs (on scaffold and tissue culture polystyrene [TCPS]) and the expression rate of neuronal markers (beta-tubulin, nestin, GFAP, and MAP-2).
Results: qPCR analysis showed that beta-tubulin (1.672 fold; P ≤ 0.0001), nestin (11.145 fold; P ≤ 0.0001), and GFAP (80.171; P ≤ 0.0001) gene expressions were higher on scaffolds compared with TCPS. Immunofluorescence analysis showed that nestin and beta-tubulin proteins were recognized in the PDMSCs differentiated on TCPS and scaffold after 7 days in the neuroinductive differentiation medium.
Conclusion: Taken together, these results delegated that PDMSCs differentiated on PLLA/PCL scaffolds are more likely to differentiate towards diversity lineages of neural cells. It proposed that PDMSCs have cell subpopulations that have the capability to be differentiated into neurogenic cells.
Keywords: Placenta-derived stem cells, Differentiation, Neural-like cells, Fibrous scaffold
Introduction
Brain and spinal cord injuries are the greatest severe difficulties in medicine and contain a diversity of neuropathology lesions.1,2 To date, some treatments have been revealed to change the development of the disease and none have effectively terminated the tissue degeneration. In the current study, stem cells were examined as a graft for neural cell substitution in neurodegenerative diseases. Stem cells are defined as undifferentiated cells with the potentiality of self-renewal and differentiation into multi-lineage cells3 that have been harvested from a varied tissue. There are several studies on the differentiation of neurons from fetal or adult neural stem cells (NSCs), human embryonic stem cells, and neural progenitor cells.4-6 However, the clinical uses of these stem cells are problematic because of the ethical concerns, limitation in the choice of lines, and tumor formation after transplantation.7 This, by no means, proposes that the NSCs and embryonic stem cells (ESCs) will not serve as an autologous cell source and transition from bench to bedside, but even studies are necessary to identify other stem cells such as mesenchymal stem cells (MSCs), with the ability to create neurons with high efficiency. MSCs appear to have the best potential for regenerative medicine and are presently used in clinical trials for a number of disorders.8-10
To date, MSCs have been isolated from different sources such as bone marrow,11 peripheral blood,12 umbilical cord blood,13 amniotic fluid,14 and Wharton’s jelly.15 However, the usage of the placental tissue-derived cells was recommended as a plentiful, ethically acceptable, and simply accessible source of cells.16,17
A previous study showed that the tissue microenvironment is a serious element in the differentiation of stem cells and straight effects on their function. Three-dimensional (3D) cultures are important in cell engineering for the achievement of mature cells18 by refining the cell-cell and cell-matrix interactions19 that directly impact cell functions.
The purpose of this study was to create the neural cells from placenta-derived mesenchymal stem cells (PDMSCs) using suitable induction reagents in the scaffold (3D) and tissue culture polystyrene (TCPS) (2D) culture systems.
Materials and Methods
Fabrication and characterization of electrospun nanofibrous PLLA/PCL scaffolds
Poly-L-lactide acid (PLLA) and polycaprolactone (PCL) were dissolved in chloroform (6% and 12 % w/w, respectively) and N, N-dimethylformamide (DMF) (Sigma, Steinheim, Germany) solution. Next, the aligned nanofibrous PLLA/PCL scaffolds were created by an electrospinning machine (distance: 200 mm, flow rate: 0.1 mL/h, the voltage: 24 kv). The Fourier-transform infrared spectroscopy (FTIR) was used to ascertain the chemical properties of PLLA/PCL nanofibrous scaffolds.
Isolation, cell surface, and multipotent differential analysis of PDMSCs
The placental tissue was achieved after cesarean sections (from a healthy donor) with the consent forms considering the ethical principles of Tehran University of Medical Sciences (TUMS) guidelines. A piece of placental tissue was isolated, minced, washed (with a phosphate-buffered saline solution), and digested in enzymatic treatment (0.1% collagenase IV; Sigma Aldrich, St. Louis, MO) for 2–3 hours in 37°C. The cell suspension was divided by a sterile pipette from the rest of the undigested tissue and cultivated in a plastic culture dish with DMEM complemented with 10% FBS and 2% antibiotic (penicillin/streptomycin) in incubator environment (37°C, 5% CO2). Then, the non-adherent cells were detached from the culture dish by trypsin–EDTA (0.25%) solvent, was applied for subculturing up to 3 passages to reach enough cells for carrying out the next experiments.20 To confirm the MSCs identity, isolated cells (in the 3 passages) were analyzed for the expression of surface antigens by using antibodies against human CD105, CD73, CD90, CD44, CD34, and CD45 surface antigens(eBioscience, San Diego, CA) by Flow cytometry. To confirm the differentiation potential, the cells were cultured in osteogenic (containing 50 mg ascorbic acid bi-phosphate (Sigma), 100 nM dexamethasone (Sigma), and 10 mM beta-glycerol-phosphate (Merck, Germany)) or adipogenic medium (containing 0.5 mM 3- isobutyl-1-methylxanthine and 250 nM dexamethasone; Sigma). After 21 days, specific staining (Alizarin Red S and Oil Red O) were used for the evaluation of osteogenic and adipogenic differentiation potential of PDMSCs, respectively.20
In vitro differentiation of cells on PLLA/PCL scaffold
The PLLA/PCL scaffold was positioned in alcohol for sterilization and the cells were seeded on them. For neural differentiation, the cells were treated in an induction medium (DMEM (low glucose) added by 10 mM retinoic acid (RA, Sigma), 10 mM Forskolin and 0.5 mM IBMX for 7 days on PLLA/PCL scaffold and TCPS. Next, the differentiated cells were used for qPCR and Immunofluorescence assay.
MTT assay
The viability of cells on the scaffold (3D) in comparison with 2D culture group was determined using MTT assay. The 1.5 × 103 cells were cultured in 24-well cell culture plate and on the scaffold at 37°C for 3 and 5 days. Then, MTT solution (5 mg/mL) was added and after 3 hours, the formazan crystals were solubilized using dimethylsulfoxide. The incubated suspension was read at 570 nm using a microplate reader (ELX800; BioTeK, Winooski, VT).
Immunostaining analysis
The cells (on TCPS and Scaffold) were fixed (4% paraformaldehyde), permeabilized (with 0.5% Triton X-100), incubated with primary antibodies for nestin (Millipore) and beta-tubulin III (Sigma) at 4°C overnight, and subsequently incubated with FITC-conjugated IgG (Santa Cruz Biotechnology) at room temperature for 1 hour. For nuclear staining, the cells were incubated with diaminobenzidine (DAB) solution (Sigma Chemical Co.) for 30 seconds.
qPCR analysis
The total RNA was extracted, their quantification and purity were determined, and cDNA was synthesized by the PrimeScript 1st strand synthesis Kit (Takara. Japan). Real-time PCR was done with an Applied BiosystemsTM System (Life Technologies Corporation, USA). All genes were subjected to 40 cycles, which consisted of initial denaturation at 95°C for 15 minutes, followed by 40 cycles of denaturation at 95°C for 15 seconds, annealing at 60°C for 30 seconds, and a final extension at 72°C for 30 seconds. The related specific gene primers are recorded in Table 1.
Table 1. Neural gene primers used for q-PCR
|
Genes
|
Primer sequences
|
Size (bp)
|
Nestin |
F: 5´ GAA GGT GAA GGG CAA ATC TG 3´ R: 5´ CCT CTT CTT CCC ATA TTT CCT G 3´ |
96 |
GFAP |
F: 5´ GCA GAC CTT CTC CAA CCT G 3´ R: 5´ ACT CCT TAA TGA CCT CTC CAT C 3´ |
127 |
MAP2 |
F: 5´ AGT TCC AGC AGC GTG ATG 3´ R: 5´ CAT TCT CTC TTC AGC CTT CTC 3´ |
97 |
TUBB3 |
F: 5´ TGG AGT GAG AGG CAG GTG 3´ R: 5´ GTG TCG GCA GCA AGA TGG 3´ |
76 |
GAPDH |
F: 5´ GTGAACCATGAGAAGTATGACAAC 3´ R: 5´ CATGAGTCCTTCCACGATACC 3´ |
123 |
Statistical Analysis
Statistical analysis was done by using Graph pad prism 6 app and Rest software for MTT assay and qRT PCR, respectively. Two-way ANOVA was performed for MTT assay analysis.
Results
Isolation and characterization of cells from the placental tissue
The isolated cells had a spindle-shaped morphology and after osteogenic and adipogenic treatment showed the differentiation into adipocytes and osteocytes. Moreover, Flow cytometry result indicated that the cells are positive for CD44, CD73, CD105, and CD90, and negative for CD45 and CD34.20
The FTIR results showed the presence of both polymers PLLA and PCL in the hybrid nanofibrous scaffold. The peaks at 2948 and 2868 cm-1 are due to the C–H stretching, at 1758 and 1735cm-1 match up to the C=O bonding, and at 1183 cm-1is due to the C–O bending (Fig. 1).
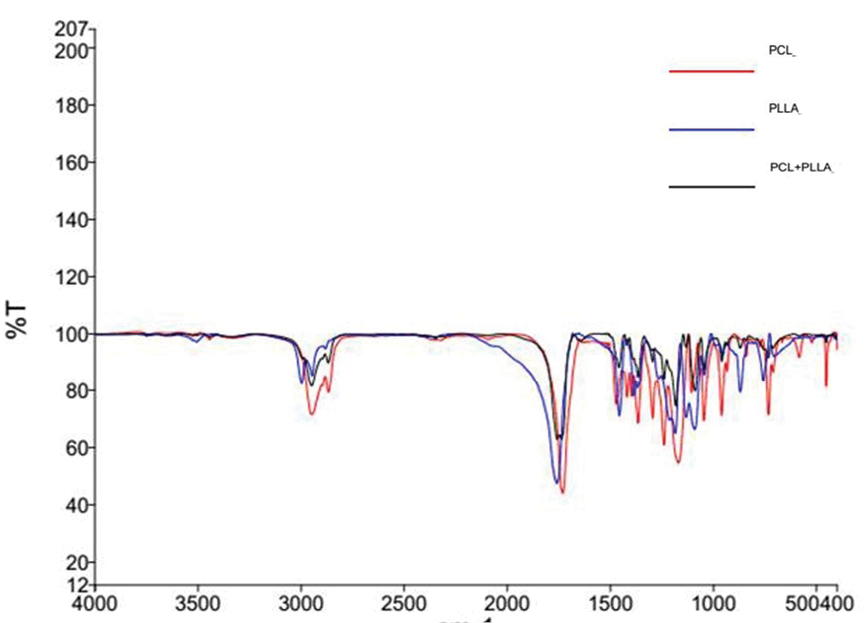
FT-IR analysis of PLLA/PCL scaffold.
MTT assay
The viability of isolated cells on the PLLA/PCL scaffold was studied after 3 and 5 days of cultivating. The MTT result showed that the proliferation of cells on the scaffold was higher than those on TCPS (Fig. 2).
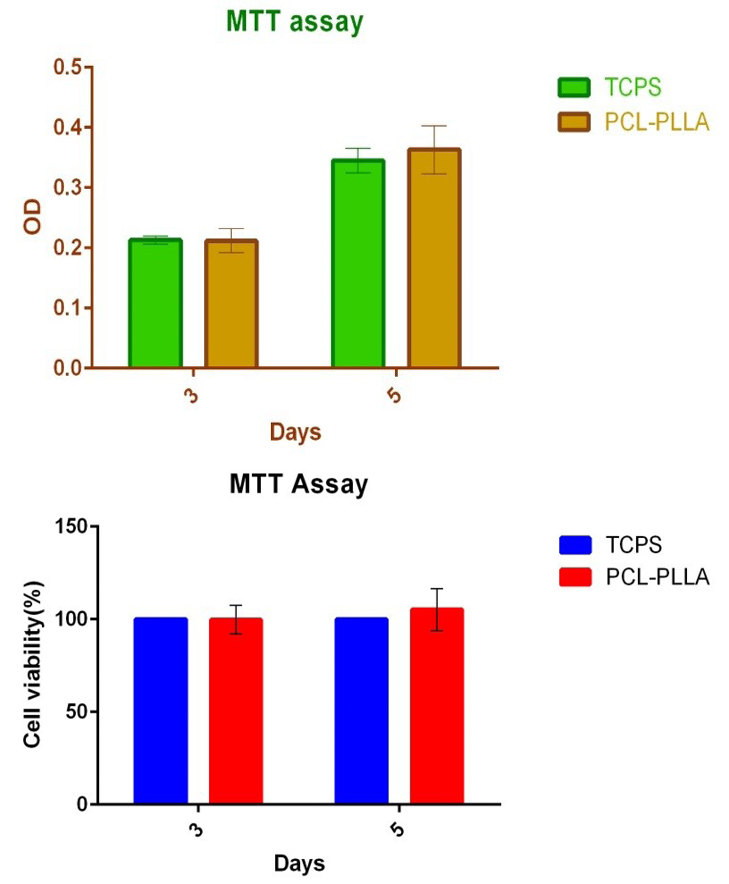
MTT assay analysis.
Neural differentiation of cells
To reveal the neural differentiation potential of the PMSCs, the cells were seeded on TCPS and fibrous scaffold in the neuroinductive medium. Morphological variations in the cells were detected during differentiation (Fig. 3). Next, circle and multipolar morphologies with cytoplasmic branches were detected 5, 6, and 7 days after induction in PDMSCs (Fig. 3). The electron microscopy images indicated that the cells were attached and produced neurite outgrowth on scaffolds (Fig. 4). After 7 days, we studied the expression of neural genes and protein using qPCR and immunofluorescence. Immunofluorescence showed that nestin and beta-tubulin proteins were recognized in the PDMSCs differentiated on PLLA/PCL scaffold and TCPS after induction in neurogenic medium (Fig. 5).
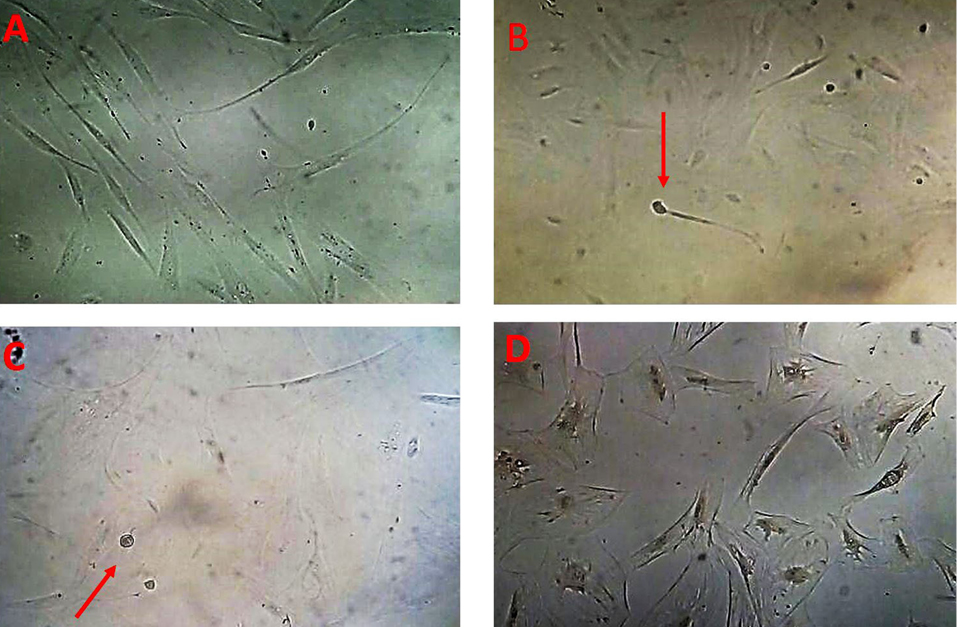
Cell morphological changes in PDMSCs during induction.
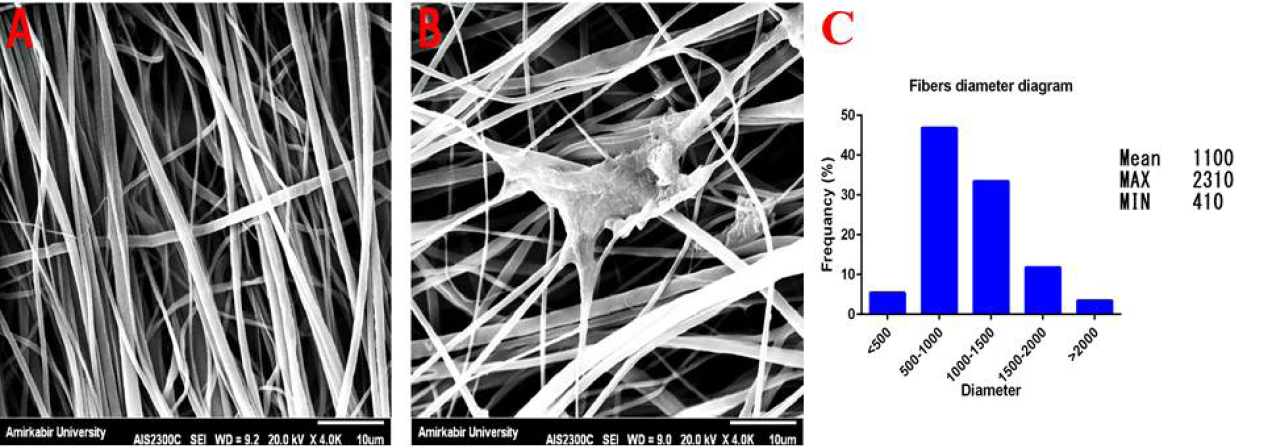
SEM images of scaffolds. The PLLA/PCL scaffold (A) and PDMSCs differentiated 7 days after induction (B) on it. C) The frequency fiber diameter of scaffold.
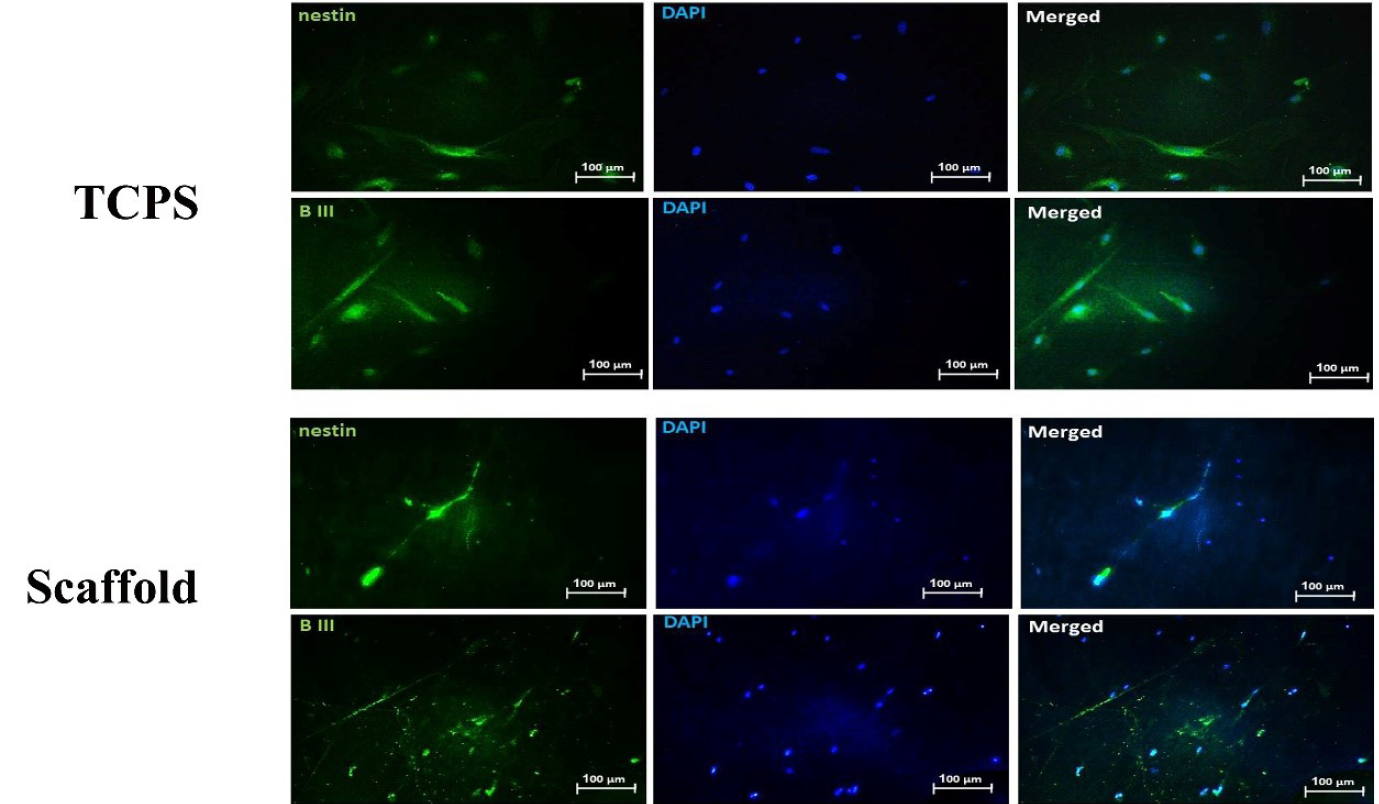
Immunofluorescent imaging of cells. The seeded cells on scaffold and TCPS were retained in neural induction medium for 7 days and examined for beta tubulin and nestin proteins expression. Furthermore, to visualize nuclei, the cells were co-stained with 4,6-diamidino-2-phenylindole (blue).
qPCR analysis
The expression of four genes on the PLLA/PCL scaffold and TCPS were examined by qPCR (Fig. 6). As presented in Fig. 6, qPCR showed that the expression levels of beta tubulin (1.672 fold; P ≤ 0.0001), nestin (11.145 fold; P ≤ 0.0001), and GFAP (80.171; P ≤ 0.0001) genes were higher on scaffold related to TCPS.
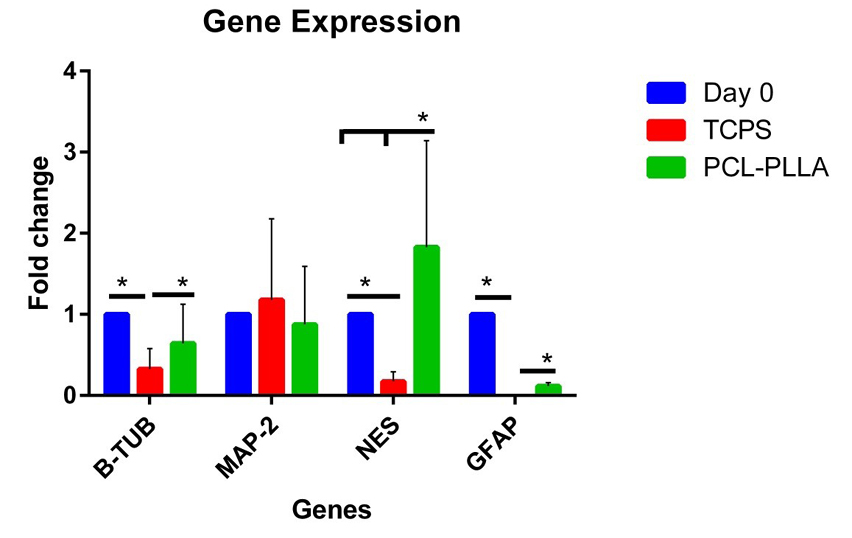
The qPCR analysis of neural genes. The expression of four genes in day 0 (control) and in day 7 of PDMSCs on TCPS and scaffold were examined. The Rest software was used for statistical analysis of data.
Discussion
Stem cell therapy is another methodology that has been targeted for the restoration of the CNS damaged.21 MSCs have been intensively studied for stem cell-based transplantation therapy and repairing spinal cord injury11,22 due to the easy availability, low immunogenicity,23,24 paracrine and immune modulatory effects.25 Moreover, scaffold transplantation supported tissue repair by reducing the cavity size at the lesion site.26 This work was expected to study appropriate stem cell and biomaterial interactions for neural tissue engineering. To date, numerous stem cells have been studied for neurological disorders, such as ESCs5,27,28 and MSCs. However, the complications of ESCs such as ethical matters and tumorigenic capability led many investigators to investigate alternate cell sources for the treatment of diseases.
In this work, we studied the neural differentiation of MSCs, isolated from placental tissue, which are waste after childbirth; then they are simply available and cause no ethical concerns.29,30 The PDMSCs have a spindle-shaped morphology with multiple mesodermal differentiation.20 We designed to explore whether MSCs isolated from human placental tissue can differentiate into neural cells using induction medium including RA, cAMP, and forskolin supplementation as a neural induction factor, in one step of induction protocol. The PDMSCs have shown their capability in differentiating into neural-like cells, with this characteristic being identified through morphology, q-PCR, and Immunostaining. In this work, the PDMSCs expressed neuronal genes and proteins after treatment with DMEM supplemented with retinoic acid, forskolin, and IBMX for 1 week. The q-PCR analysis showed that neurogenic genes including MAP-2, beta tubulin, and nestin were expressed in PDMSCs on TCPS culture. Furthermore, Immunostaining results revealed that beta-tubulin and nestin proteins were expressed in PDMSCs.
Research projects on tissue engineering recognize that the scaffold properties may impact the fate decision of the stem cells.31,32 Number studies are motivated on the improvement of fibrous scaffolds to conduit the lesion cavities and provide tissue renovation.33-35 A perfect scaffold would contain the properties such as good biocompatibility, low immunogenicity, appropriate biodegradability once graftedin vivo, appropriate properties for adhesion and axonal renewal of the cells, and the capability of offering guidance to the regenerating axons.35 Jakobsson et al36 showed that PCL nanoscaffolds displayed excellent cell survival and raised neuronal differentiation, as related to cultures grown in 2D dishes.37 Moreover, the PLLA scaffold can encourage the neural differentiation of MSCs by supporting neuron differentiation and neurite outgrowth in order to mimic a natural extracellular matrix of natural collagen throughout the scaffold.38 However the hybrid scaffolds have an overall exceptional beneficial over other fibers.39,40 In our study, we established a PLLA/PCL hybrid scaffold for neural differentiation of PDMSCs. Numerous studies have revealed the impacts of electrospun scaffolds of biomaterials41 to support the neural differentiation of stem cells. In the present study, we studied the impact of PLLA/PCL nanofibrous scaffold, invented by electrospinning techniques, on the neural differentiation of PDMSCs. In this study, after exposure of PDMSCs to neural induction media, the qPCR analysis showed that the neurogenic genes including beta-tubulin, MAP-2, nestin, and GFAP were expressed in seeded cells on the fibrous scaffold. The scaffolds have 3D features that recapitulate the innate microenvironment of the cells and simulate some features of the natural extracellular matrix of tissue.42 In this study, the PDMSCs seeded on the scaffolds can modify the expression of the neural genes. qPCR analysis showed that in PDMSCs differentiated on the scaffolds, neural genes such as beta-tubulin, nestin (neuro-ectoderm marker),43 and GFAP (glial lineage marker)44 were expressed at higher levels compared to cells differentiated on TCPS dishes. However, it is possible that the interaction between the cells (PDMSCs) and the fibrous scaffold (PLLA/PCL) can raise the expression of the specific neuronal markers.45
Conclusion
Taken together, these results indicated that the PDMSCs differentiated on PLLA/PCL scaffolds are more likely to differentiate towards diverse lineages of neural cells. It proposed that PDMSCs have a subpopulation of the cells that are able to be differentiated into neurogenic cells.
Acknowledgments
The authors would like to thank Dr. Naser Ahmadbeigi, manager of SABZ Company, who was kindly gifted source of stem cells (PDMSCs) that were isolated and characterized in his ZABZ Company.
Funding sources
The study was financially supported by Deputy of the Research and Technology, ZUMS (Grant No: A-10-420-8).
Ethical statement
This work was approved by Zanjan University of Medical Sciences (ZUMS) under Ethical No: ZUMS.REC.1398.98.
Competing interests
There is no conflict of interests in this study.
Authors’ contribution
FRS: Draft preparation, Writing and reviewing, Data handling. SN: Conceptualization, experiments design, study validation, writing and reviewing, data analysis. AR: Data handling, Data analysis, Writing. ADO: Conceptualization, Trials design, Statistical analysis, Facility of study materials and equipment, Study justification, Supervision, Writing and reviewing.
References
- Sun T, Ye C, Wu J, Zhang Z, Cai Y, Yue F. Treadmill step training promotes spinal cord neural plasticity after incomplete spinal cord injury. Neural Regen Res 2013; 8: 2540-7. doi:10.3969/j.issn.1673-5374.2013.27.005. [Crossref]
- Liu J, Yang X, Jiang L, Wang C, Yang M. Neural plasticity after spinal cord injury. Neural Regen Res 2012; 7: 386-91. doi:10.3969/j.issn.1673-5374.2012.05.010. [Crossref]
- Fuchs E, Segre JA. Stem cells: a new lease on life. Cell 2000; 100: 143-55. doi:10.1016/s0092-8674(00)81691-8. [Crossref]
- Buytaert-Hoefen KA, Alvarez E, Freed CR. Generation of tyrosine hydroxylase positive neurons from human embryonic stem cells after coculture with cellular substrates and exposure to GDNF. Stem Cells 2004; 22: 669-74. doi:10.1634/stemcells.22-5-669. [Crossref]
- Perrier AL, Tabar V, Barberi T, Rubio ME, Bruses J, Topf N, et al. Derivation of midbrain dopamine neurons from human embryonic stem cells. Proc Natl Acad Sci U S A 2004; 101: 12543-8. doi:10.1073/pnas.0404700101. [Crossref]
- Zeng X, Cai J, Chen J, Luo Y, You ZB, Fotter E, et al. Dopaminergic differentiation of human embryonic stem cells. Stem Cells 2004; 22: 925-40. doi:10.1634/stemcells.22-6-925. [Crossref]
- Lie DC, Dziewczapolski G, Willhoite AR, Kaspar BK, Shults CW, Gage FH. The adult substantia nigra contains progenitor cells with neurogenic potential. J Neurosci 2002; 22: 6639-49. doi:20026700. [Crossref]
- Alhadlaq A, Mao JJ. Mesenchymal stem cells: isolation and therapeutics. Stem Cells Dev 2004; 13: 436-48. doi:10.1089/scd.2004.13.436. [Crossref]
- Phinney DG, Isakova I. Plasticity and therapeutic potential of mesenchymal stem cells in the nervous system. Curr Pharm Des 2005; 11: 1255-65. doi:10.2174/1381612053507495. [Crossref]
- Schwarz EJ, Alexander GM, Prockop DJ, Azizi SA. Multipotential marrow stromal cells transduced to produce L-DOPA: engraftment in a rat model of Parkinson disease. Hum Gene Ther 1999; 10: 2539-49. doi:10.1089/10430349950016870. [Crossref]
- Tropel P, Platet N, Platel JC, Noel D, Albrieux M, Benabid AL, et al. Functional neuronal differentiation of bone marrow-derived mesenchymal stem cells. Stem Cells 2006; 24: 2868-76. doi:10.1634/stemcells.2005-0636. [Crossref]
- Kim S, Honmou O, Kato K, Nonaka T, Houkin K, Hamada H, et al. Neural differentiation potential of peripheral blood- and bone-marrow-derived precursor cells. Brain Res 2006; 1123: 27-33. doi:10.1016/j.brainres.2006.09.044. [Crossref]
- El-Badri NS, Hakki A, Saporta S, Liang X, Madhusodanan S, Willing AE, et al. Cord blood mesenchymal stem cells: Potential use in neurological disorders. Stem Cells Dev 2006; 15: 497-506. doi:10.1089/scd.2006.15.497. [Crossref]
- Prusa AR, Marton E, Rosner M, Bettelheim D, Lubec G, Pollack A, et al. Neurogenic cells in human amniotic fluid. Am J Obstet Gynecol 2004; 191: 309-14. doi:10.1016/j.ajog.2003.12.014. [Crossref]
- Mitchell KE, Weiss ML, Mitchell BM, Martin P, Davis D, Morales L, et al. Matrix cells from Wharton's jelly form neurons and glia. Stem Cells 2003; 21: 50-60. doi:10.1634/stemcells.21-1-50. [Crossref]
- Chan J, Kennea NL, Fisk NM. Placental mesenchymal stem cells. Am J Obstet Gynecol 2007; 196: e 18; author reply e-9. doi:10.1016/j.ajog.2006.08.002. [Crossref]
- Portmann-Lanz CB, Schoeberlein A, Huber A, Sager R, Malek A, Holzgreve W, et al. Placental mesenchymal stem cells as potential autologous graft for pre- and perinatal neuroregeneration. Am J Obstet Gynecol 2006; 194: 664-73. doi:10.1016/j.ajog.2006.01.101. [Crossref]
- Takeuchi H, Nakatsuji N, Suemori H. Endodermal differentiation of human pluripotent stem cells to insulin-producing cells in 3D culture. Sci Rep 2014; 4: 4488. doi:10.1038/srep04488. [Crossref]
- Grayson WL, Ma T, Bunnell B. Human mesenchymal stem cells tissue development in 3D PET matrices. Biotechnol Prog 2004; 20: 905-12. doi:10.1021/bp034296z. [Crossref]
- Kamalabadi-Farahani M, Vasei M, Ahmadbeigi N, Ebrahimi-Barough S, Soleimani M, Roozafzoon R. Anti-tumour effects of TRAIL-expressing human placental derived mesenchymal stem cells with curcumin-loaded chitosan nanoparticles in a mice model of triple negative breast cancer. Artif Cells Nanomed Biotechnol 2018; 46: S1011-S21. doi:10.1080/21691401.2018.1527345. [Crossref]
- Okano H. Neural stem cells and strategies for the regeneration of the central nervous system. Proc Jpn Acad Ser B Phys Biol Sci 2010; 86: 438-50. doi:10.2183/pjab.86.438. [Crossref]
- Dong L, Hao H, Han W, Fu X. The role of the microenvironment on the fate of adult stem cells. Sci China Life Sci 2015; 58: 639-48. doi:10.1007/s11427-015-4865-9. [Crossref]
- Di Nicola M, Carlo-Stella C, Magni M, Milanesi M, Longoni PD, Matteucci P, et al. Human bone marrow stromal cells suppress T-lymphocyte proliferation induced by cellular or nonspecific mitogenic stimuli. Blood 2002; 99: 3838-43. doi:10.1182/blood.v99.10.3838. [Crossref]
- Jiang Y, Jahagirdar BN, Reinhardt RL, Schwartz RE, Keene CD, Ortiz-Gonzalez XR, et al. Pluripotency of mesenchymal stem cells derived from adult marrow. Nature 2002; 418: 41-9. doi:10.1038/nature00870. [Crossref]
- D'Souza N, Rossignoli F, Golinelli G, Grisendi G, Spano C, Candini O, et al. Mesenchymal stem/stromal cells as a delivery platform in cell and gene therapies. BMC Med 2015; 13: 186. doi:10.1186/s12916-015-0426-0. [Crossref]
- Hong LTA, Kim YM, Park HH, Hwang DH, Cui Y, Lee EM, et al. An injectable hydrogel enhances tissue repair after spinal cord injury by promoting extracellular matrix remodeling. Nat Commun 2017; 8: 533. doi:10.1038/s41467-017-00583-8. [Crossref]
- Reubinoff BE, Itsykson P, Turetsky T, Pera MF, Reinhartz E, Itzik A, et al. Neural progenitors from human embryonic stem cells. Nat Biotechnol 2001; 19: 1134-40. doi:10.1038/nbt1201-1134. [Crossref]
- Zhang SC, Wernig M, Duncan ID, Brustle O, Thomson JA. In vitro differentiation of transplantable neural precursors from human embryonic stem cells. Nat Biotechnol 2001; 19: 1129-33. doi:10.1038/nbt1201-1129. [Crossref]
- Yen BL, Huang HI, Chien CC, Jui HY, Ko BS, Yao M, et al. Isolation of multipotent cells from human term placenta. Stem Cells 2005; 23: 3-9. doi:10.1634/stemcells.2004-0098. [Crossref]
- Fukuchi Y, Nakajima H, Sugiyama D, Hirose I, Kitamura T, Tsuji K. Human placenta-derived cells have mesenchymal stem/progenitor cell potential. Stem Cells 2004; 22: 649-58. doi:10.1634/stemcells.22-5-649. [Crossref]
- Vacanti JP, Langer R. Tissue engineering: the design and fabrication of living replacement devices for surgical reconstruction and transplantation. Lancet 1999; 354 Suppl 1: SI32-4. doi:10.1016/s0140-6736(99)90247-7. [Crossref]
- Soman P, Tobe BTD, Lee JW, Winquist AM, Singec I, Vecchio KS, et al. Three-dimensional scaffolding to investigate neuronal derivatives of human embryonic stem cells. Biomed Microdevices 2012; 14: 829-38. doi:10.1007/s10544-012-9662-7. [Crossref]
- Pires LR, Pego AP. Bridging the lesion-engineering a permissive substrate for nerve regeneration. Regen Biomater 2015; 2: 203-14. doi:10.1093/rb/rbv012. [Crossref]
- Wang M, Zhai P, Chen X, Schreyer DJ, Sun X, Cui F. Bioengineered scaffolds for spinal cord repair. Tissue Eng Part B Rev 2011; 17: 177-94. doi:10.1089/ten.TEB.2010.0648. [Crossref]
- Straley KS, Foo CW, Heilshorn SC. Biomaterial design strategies for the treatment of spinal cord injuries. J Neurotrauma 2010; 27: 1-19. doi:10.1089/neu.2009.0948. [Crossref]
- Jakobsson A, Ottosson M, Zalis MC, O'Carroll D, Johansson UE, Johansson F. Three-dimensional functional human neuronal networks in uncompressed low-density electrospun fiber scaffolds. Nanomedicine 2017; 13: 1563-73. doi:10.1016/j.nano.2016.12.023. [Crossref]
- Jahani H, Jalilian FA, Wu CY, Kaviani S, Soleimani M, Abassi N, et al. Controlled surface morphology and hydrophilicity of polycaprolactone toward selective differentiation of mesenchymal stem cells to neural like cells. J Biomed Mater Res A 2015; 103: 1875-81. doi:10.1002/jbm.a.35328. [Crossref]
- Yang F, Murugan R, Ramakrishna S, Wang X, Ma YX, Wang S. Fabrication of nano-structured porous PLLA scaffold intended for nerve tissue engineering. Biomaterials 2004; 25: 1891-900. doi:10.1016/j.biomaterials.2003.08.062. [Crossref]
- Kenry, Lim CT. Nanofiber technology: current status and emerging developments. Prog Polym Sci 2017; 70: 1-17. doi:https://doi.org/10.1016/j.progpolymsci.2017.03.002. [Crossref]
- Tambralli A, Blakeney B, Anderson J, Kushwaha M, Andukuri A, Dean D, et al. A hybrid biomimetic scaffold composed of electrospun polycaprolactone nanofibers and self-assembled peptide amphiphile nanofibers. Biofabrication 2009; 1: 025001. doi:10.1088/1758-5082/1/2/025001. [Crossref]
- Bini TB, Gao S, Xu X, Wang S, Ramakrishna S, Leong KW. Peripheral nerve regeneration by microbraided poly(L-lactide-co-glycolide) biodegradable polymer fibers. J Biomed Mater Res A 2004; 68: 286-95. doi:10.1002/jbm.a.20050. [Crossref]
- Larsen M, Artym VV, Green JA, Yamada KM. The matrix reorganized: extracellular matrix remodeling and integrin signaling. Curr Opin Cell Biol 2006; 18: 463-71. doi:10.1016/j.ceb.2006.08.009. [Crossref]
- Livesey FJ, Young TL, Cepko CL. An analysis of the gene expression program of mammalian neural progenitor cells. Proc Natl Acad Sci U S A 2004; 101: 1374-9. doi:10.1073/pnas.0307014101. [Crossref]
- Neeley WL, Redenti S, Klassen H, Tao S, Desai T, Young MJ, et al. A microfabricated scaffold for retinal progenitor cell grafting. Biomaterials 2008; 29: 418-26. doi:10.1016/j.biomaterials.2007.10.007. [Crossref]
- Ilie I, Ilie R, Mocan T, Bartos D, Mocan L. Influence of nanomaterials on stem cell differentiation: designing an appropriate nanobiointerface. Int J Nanomedicine 2012; 7: 2211-25. doi:10.2147/IJN.S29975. [Crossref]