Bioimpacts. 14(2):27829.
doi: 10.34172/bi.2023.27829
Original Article
Introduction of a new recombinant vaccine based on GRP78 for breast cancer immunotherapy and evaluation in a mouse model
Hamed Zare Formal analysis, Investigation, Methodology, Resources, Software, Validation, Writing – review & editing, 1 
Hamid Bakherad Conceptualization, Funding acquisition, Investigation, Methodology, Project administration, Resources, Supervision, Validation, Writing – original draft, 2, 3, * 
Arman Nasr Esfahani Investigation, 2
Mohamad Norouzi Investigation, Resources, 2
Hossein Aghamollaei Investigation, 4
Seyed Latif Mousavi Gargari Methodology, Validation, 5
Fatemeh Mahmoodi Investigation, Writing – review & editing, 6
Mahdi Aliomrani Methodology, Validation, 7
Walead Ebrahimizadeh Investigation, Writing – review & editing, 8
Author information:
1Recombinant Proteins Department, Breast Cancer Research Center, Motamed Cancer Institute, ACECR, Tehran, Iran
2Department of Pharmaceutical Biotechnology, Faculty of Pharmacy and Pharmaceutical Sciences, Isfahan University of Medical Sciences, Isfahan, Iran
3Pharmaceutical Sciences Research Center, Isfahan University of Medical Sciences, Isfahan, Iran
4Chemical Injuries Research Center, Systems Biology and Poisonings Institute, Baqiyatallah University of Medical Sciences, Tehran, Iran
5Department of Biology, Faculty of Basic Science, Shahed University, Tehran, I.R. Iran
6Department of Biology, College of Sciences, Shiraz University, Shiraz, Iran
7Department of Pharmacology and Toxicology, Isfahan Pharmaceutical Science Research Center, Faculty of Pharmacy and Pharmaceutical Sciences, Isfahan University of Medical Sciences, Isfahan, Iran
8Department of Surgery, Division of Urology, McGill University, and the Research Institute of the McGill University Health Centre (RI MUHC), Montreal, Quebec, Canada
Abstract
Introduction:
Breast cancer is one of the most prevalent malignancies in women. Several treatment options are available today, including surgery, chemotherapy, and radiotherapy. Immunotherapy, as a highly specific therapy, involves adaptive immune responses and immunological memory. In our present research, we used the recombinant C-terminal domain of the GRP78 (glucose- regulated protein 78) protein to induce an immune response and investigate its therapeutic impact in the 4T1 breast cancer model.
Methods:
BALB/c mice were immunized with the cGRP78 protein. The humoral immune response was assessed by ELISA. Then, BALB/c mice were injected subcutaneously with 1×106 4T1 tumor cells. Subsequently, tumor size and survival rate measurements, MTT, and cytokine assays were performed.
Results:
The animals receiving the cGRP78 vaccine showed significantly more favorable survival and slower tumor growth rates compared with unvaccinated tumor-bearing mice as the negative control mice. Circulating levels of tumoricidal cytokines such as IFNγ were higher, whereas tolerogenic cytokines such as IL-2, 6, and 10 either did not increase or had a decreasing trend in mice receiving cGRP78.
Conclusion:
cGRP78 vaccines generated potent immunotherapeutic effects in a breast cancer mouse model. This novel strategy of targeting the GRP78 protein can promote the development of cancer vaccines and immunotherapies for breast cancer malignancies.
Keywords: cGRP78, Breast cancer, Vaccine, Immunotherapy, Metastasis
Copyright and License Information
© 2024 The Author(s).
This work is published by BioImpacts as an open access article distributed under the terms of the Creative Commons Attribution Non-Commercial License (
http://creativecommons.org/licenses/by-nc/4.0/). Non-commercial uses of the work are permitted, provided the original work is properly cited.
Introduction
Glucose-regulated protein 78 (GRP78) is a member of the HSP70 (heat shock protein 70) super family, which is found in the endoplasmic reticulum (ER) lumen. The main functions of GRP78 are protein assembly, folding, and transfer to its functional site in the ER membrane. Other tasks of GRP78 include targeting incorrectly folded proteins for proteasomal degradation and regulating calcium homeostasis in normal cells.1,2
Multiple studies have reported surface overexpression of GRP78 in many cancer types. Studies show GRP78 expression is highly increased in proliferating cancer cells and tumor-related endothelium. This is in response to stress caused by severe lactic acidosis, hypoxia, and glucose starvation (tumor microenvironment characteristics). In cancer cells, ER membrane-spanning GRP78 is transported to the cell surface. This phenomenon is unique to cancer and rarely observed in normal cells. The level of expression and surface translocation is often correlated with the rate of proliferation, malignancy, anti-apoptotic activity, drug resistance, and metastasis.2-4 Many cancer types, such as breast cancer, hepatoma, melanoma, pancreatic cancer, and osteosarcoma, have elevated GRP78 surface expression. These findings indicate cell surface GRP78 is a promising cancer cell-specific biomarker and a beneficial target for cancer treatment.5,6
Metastatic cancer cells express high levels of GRP78 on the cell surface, and this high expression is associated with lymph node metastasis.7 Specifically, in breast cancer (BC), it was found that high levels of cell surface GRP78 promote high phosphorylation rates of STAT3 and boost cancer cell growth and migration. Cell surface GRP78 can function either to activate apoptotic or anti-apoptotic signaling pathways in cancer cells.8 GRP78 autoantibodies such as C38 that target the C-terminus domain of the protein can activate the P53 apoptotic signaling pathway and start apoptosis, resulting in tumor growth inhibition. This further highlights the importance of targeting GRP78 to inhibit the growth and metastasis of cancer cells.9 The C-terminal of GRP78 is a convenient target for developing inhibitory antibodies or vaccines in cancer patients.10
BC is the most common malignancy in women and the second leading cause of cancer death worldwide. One in nine women will develop BC in their lifetime, and nearly 14.5 million women lived with BC in 2014. This figure is expected to rise to almost 19 million by 2024.11 Therapeutic options for BC include chemotherapy, radiotherapy, surgery, hormone therapy, and immunotherapy that block tumor cell growth, proliferation, and invasiveness. However, these treatments have little effect on clinical practice. Anticancer vaccine therapy is more tumor-specific and less harmful to normal cells.12,13 Anticancer vaccines can eliminate cancer cells by activating the innate and antibody-mediated immune responses. Following the injection of the vaccine, the immune response is activated by antigen phagocytosis and its processing by antigen-presenting cells (APCs). APCs insert antigens into major histocompatibility complex (MHC) class I to activate T-cell-mediated cytotoxicity and inert antigen into MHC class II to activate T-helper cell response and humoral immunity.12-14
In our previous studies, we generated the C-terminal domain of GRP78 via in silico tools. We further accredit this antigen based on the experimental data through ELISA and CD analysis. The results showed that the 3D structure of the recombinant protein is very similar to the intact protein structure. This truncated protein could be a good candidate for an anti-cancer vaccine.10 Unlike most BC vaccines that are against HER2, which covers a small proportion of patients.15 The cGRP78-based vaccine can be used for all cancers. This study aims to develop a cancer vaccine based on cGRP78 and evaluate its efficacy in a breast cancer model.
Materials and Methods
Cell lines and mice
The 4T1 cell line was prepared from the cell bank of the Pasteur Institute of Iran and cultured in standard cell culture conditions in the DMEM medium (Gibco, USA) enriched with 10% fetal bovine serum (FBS) (Gibco, USA). The cells were passaged every two days to reach the number of cells required for tumorigenesis.
The 4-week-old BALB/c mice used in this study were purchased from Royan Laboratory Animal Center of Isfahan and were kept in standard conditions. The conditions and steps of working with laboratory animals were performed according to the Animal Welfare Guideline, and the proposal for this research was approved by the Institutional Ethical Committee and Research Advisory Committee of the Isfahan University of Medical Sciences in Iran (NO:1398.846).
Preparation of cGRP78
Cloning and expression of the recombinant cGRP78 protein were performed in our previous study.10 The results showed that this truncated protein could be used as a vaccine. The length of the cGRP78 protein gene was 690 bp and was cloned in the pET22b vector. In summary, the production steps of the recombinant cGRP78 protein include a culture of E. coli T7 shuffle (as the host) and induction of expression by Isopropyl ß-D-1-thiogalactopyranoside (IPTG) (Merck, Germany) at 30 °C for 16 hours. The production of recombinant cGRP78 protein was evaluated by sodium dodecyl sulfate-polyacrylamide gel electrophoresis (SDS-PAGE), and purification was performed by the Ni-NTA resin column by the native method. The recombinant protein was confirmed by ELISA with an anti-cGRP78 antibody.
Mouse immunization with cGRP78
To investigate the performance of cGRP78 recombinant protein as an anti-breast cancer vaccine, 3-4 weeks old male BALB/c mice were randomly divided into two test and control groups. After a week of adaptation of the mice to the environment, vaccine injections were started in the test and control groups. Vaccines were administered via subcutaneous and peritoneal injections in the left flank. For the first time, 50 μg cGRP78/mice in the test group and PBS in the control group with complete Freund's adjuvant were injected. Two other boosters were injected ten days apart, similar to the first injection, except Freund's complete adjuvant was replaced with Freund's incomplete adjuvant. Before each vaccine injection, blood was collected from four anesthetized mice via a capillary tube in the left eye in the test and control groups. One week after finishing the last vaccine booster, four mice from each group were sacrificed to isolate the spleen for cytokine and cell proliferation tests.
Detection of antibody response to cGRP78 by ELISA
The reaction of the humoral immune system of mice vaccinated with cGRP78 was measured by the enzyme-linked immunosorbent assay (ELISA) technique. Briefly, 96-well plates with 5 μg/well of cGRP78 protein were coated in the coating buffer (carbonate bicarbonate buffer), and after washing, 5% skim milk (Merck, Germany) was used to block the wells. Serial dilutions were prepared from the sera of immunized and control mice (1:100, 1:200, 1:500, and 1:1000) and exposed to cGRP78 for one hour with triplicate repetitions. After washing, HRP (horseradish peroxidase) conjugated goat anti-mouse IgG (Sigma-Aldrich, USA) with a dilution of 1:50 000 was added in the wells, and TMB (3, 3’, 5, 5’tetramethylbenzidine) (Merck, Germany) was used to track the bound antibodies. At the end of the reaction, after stopping the reaction with H2SO4, absorbance was measured at 450 nm with an Elisa Reader (Bio-Rad, model 680, California, USA).
Cytokine assay and splenocyte proliferation
Splenocytes from sacrificed mice were isolated from vaccinated and control mice under sterile conditions, and their cells were extracted. Red blood cells were removed with RBC lysis buffer, and the rest of the cells were cultured in Roswell Park Memorial Institute medium (RPMI) (Gibco, USA) medium supplemented with 10% FBS. 2×106 cells from splenocytes of the vaccinated and control groups were cultured in a 24-well plate with 20 μg/mL cGRP78 as a stimulating antigen for 72 hours. IL-2 and IFNγ were evaluated to measure the growth stimulation of cGRP78 antigen on T helper cells and specific Th2 cytokines, including IL-4, IL-6, and IL-10, measured by the ELISA method and particular kits.
The growth-stimulating potency of the cGRP78 antigen on spleen lymphocytes was evaluated by the MTT test. The isolated splenocytes of the vaccinated and control groups were cultured at a density of 1×105 cells/well in a 96-well plate with 20 μg/mL of cGRP78 antigen cultured in a complete medium. After 72 hours, the MTT solution was added to the wells and incubated for 4 hours. Then the medium on the cells was removed, and DMSO was used to dissolve the formazan crystals. The amount of color produced was evaluated at a wavelength of 570 nm with an ELISA reader. All experiments were performed in triplicate.
Breast tumor model and immunotherapy
To evaluate the function of the recombinant cGRP78 protein as a breast cancer vaccine, two strategies were studied. In the first phase, the vaccine was first injected into mice in the experimental group in three boosters. The control group was injected with only PBS plus adjuvant without antigen. Tumor formation was then induced by 1×106 4T1 cells subcutaneously in the left flank of vaccinated and control BALB/c mice.
In the second phase, the vaccine's performance after tumorigenesis was evaluated. Three groups of mice were considered for this stage. The first group received the cGRP78 vaccine, the second group received the cGRP78 vaccine plus five doses of doxorubicin at a concentration of 10 mg/kg, and the third group received PBS plus adjuvant as a control group. The vaccine and control group-injected boosters were administered ten days apart. In the first injection, a complete Freund adjuvant was used, and in the other two boosts, an incomplete Freund adjuvant was used.
Tumor growth changes in the test and control groups were measured with a digital caliper once every three days, and the tumor size was calculated using the formula V (mm3) = 4 π × (L1 × L22)/3. In this formula, L1 and L2 represent the largest and smallest dimensions (mm) of the tumor mass, respectively. In this experiment, the survival rates of test and control mice were evaluated by Kaplan-Meier survival analysis. To comply with ethical considerations during the investigation, mice were sacrificed if they had any of the following symptoms: If the average diameter of the tumor exceeds 1.5 cm, body weight loss of 15% of initial weight, and mice getting sick and not being able to feed.
In vivo metastatic tumor treatment
The effect of the cGRP78 vaccine on preventing the metastasis of cancer cells was investigated in test and control groups of mice. First, two groups of BALB/c mice were injected with 5×104 4T1 cancer cells per mouse via the tail vein. Then, as mentioned in the previous step, three boosters of the cGRP78 protein were injected into the test group and PBS into the control group. After one month, the mice of both groups were sacrificed, and their spleen and lungs were evaluated visually to track the tumor nodules caused by the metastasis of cancer cells. The percentage of metastasis of 4T1 cells to the spleen and lungs of test and control mice was evaluated by weighing these organs.
Histopathology examination
Histopathological examinations were performed on the cancer tissues and organs of test and control mice. Tissues and organs were fixed in 10% formaldehyde and embedded in paraffin. Five-μm slices were prepared from the samples, and after hematoxylin and eosin (H&E) staining, histopathological examinations were performed on them. Necrosis and metastases were also determined under light microscopy by a histopathological examination expert.
Statistical analysis
All experiments were performed three times. Data obtained from different experiments were analyzed using a one-way ANOVA, and the Tukey post-test and the statistical significance level were set at P ≤ 0.05. Also, a significant difference between the two groups was found by Student’s t-tests, and the statistical significance level was set at P ≤ 0.05. All statistical analysis was performed using SPSS software (version 26.0; SPSS Inc.) and GraphPad Prism 9.
Results
Expression and purification of cGRP78
The expression of the cGRP78 protein was investigated under different conditions, and the optimal expression was obtained 16 hours after induction with 0.5 mM IPTG at 30 °C. Purification of the recombinant protein was performed under native conditions using a Ni-NTA column. Native purification conditions allow the resulting protein to retain its structure and do not require refolding. The purified 28-kDa band on SDS-PAGE shows that the cGRP78 protein was obtained with purity. The western blot technique was used to confirm the obtained protein (results not shown).
Humoral immunity
Specific antibodies against recombinant cGRP78 were induced in BALB/c mice after vaccination. As shown in Fig. 1, the antibody titer rose after each immunization and plateaued after the third dose. These results indicate that the cGRP78 protein has acceptable antigenicity and stimulates the immune system to produce antibodies.
Fig. 1.
Anti-cGRP78 IgG titer after mouse immunization: After three injections in different times, the amount of antibody produced was significantly different from the control group. **P < 0.01, ***P < 0.001
Fig. 1.
Anti-cGRP78 IgG titer after mouse immunization: After three injections in different times, the amount of antibody produced was significantly different from the control group. **P < 0.01, ***P < 0.001
Cellular immune response and Splenocyte Proliferation Induced by cGRP78
To evaluate the induction of cell-mediated immunity after injection of the cGRP78 vaccine, the proliferation ability of splenocytes exposed to the antigen was investigated. Splenocytes obtained from vaccinated mice and the control group were isolated and cultured. The proliferation of these cells in the presence of cGRP78 was measured after 72 hours using the MTT assay. The results shown in Fig. 2 confirm that the cGRP78 antigen increased the proliferation of splenocytes in vaccinated mice compared to the control group. This antigen can also stimulate the cellular immune response, besides humoral immunity, in mice.
Fig. 2.
Effects of the cGRP78 immunotherapy on the proliferation index in the splenocyte population: Proliferation of immunized cells following addition of cGRP78 antigen in comparison to non-immunized cells in a standard MTT assay. Immunized cells proliferation assay represents an increase in proliferation in comparison with non-immunized cells. *P < 0.05, **P < 0.01
Fig. 2.
Effects of the cGRP78 immunotherapy on the proliferation index in the splenocyte population: Proliferation of immunized cells following addition of cGRP78 antigen in comparison to non-immunized cells in a standard MTT assay. Immunized cells proliferation assay represents an increase in proliferation in comparison with non-immunized cells. *P < 0.05, **P < 0.01
Cytokine Assay
An assay of cytokines secreted by splenocytes in the presence of antigen showed that the cGRP78 vaccine increased IFN-γ and IL-2 secretion (upregulated) and decreased TGF-β cytokines secretion (downregulated) in vaccinated mice compared with the control group (Fig. 3). The results show that this vaccine did not have a significant effect on the production of IL-6 and IL-10 in vaccinated mice compared with the control group.
Fig. 3.
Effects of the cGRP78 immunotherapy on cytokine production in mouse splenocytes: One half of the mice in each group were euthanized 1 week after the last immunotherapy, and the splenocytes were isolated and cultured for 72 hours under the described materials and methods. Cytokine levels examination showed that immunization by cGRP78 induced a strong release of IFN-α and IL-2, while TGF-β and IL-6 decreased in comparison to the negative group. In the case of IL-6, this reduction was not statistically significant (ns). *P < 0.05
Fig. 3.
Effects of the cGRP78 immunotherapy on cytokine production in mouse splenocytes: One half of the mice in each group were euthanized 1 week after the last immunotherapy, and the splenocytes were isolated and cultured for 72 hours under the described materials and methods. Cytokine levels examination showed that immunization by cGRP78 induced a strong release of IFN-α and IL-2, while TGF-β and IL-6 decreased in comparison to the negative group. In the case of IL-6, this reduction was not statistically significant (ns). *P < 0.05
Breast tumor model and cGRP78 immunotherapy
In the first strategy, the vaccine was injected into three boosters, and then tumorigenesis was performed with 4T1 cells. A comparison of the results obtained from immunized and control mice shows that the cGRP78 vaccine, in addition to reducing tumor volume in immunized mice, increased their lifespan based on the Kaplan-Meier plot. According to the results of Figs. 4A and 4B, 31 days after tumor induction, all mice in the control group died, but 85% of the vaccinated mice were alive on this date. The average tumor volumes in the test and control groups were 3608 and 6088 mm3, respectively (P value < 0.04).
Fig. 4.
(A) Tumor volume change in vaccine and control groups: A significant difference was obtained between the vaccine and control groups by Student’s t tests (P value < 0.04). (B) Survival analysis: The Kaplan-Meier curve shows the survival of the following two groups of mice (n = 25): mice that were treated with a vaccine (n = 14) and mice that did not receive a treatment (n = 11). The curve comparison with the log-rank (Mantel-Cox) test revealed statistically significant differences between the vaccine and control groups (P = 0.0002).
Fig. 4.
(A) Tumor volume change in vaccine and control groups: A significant difference was obtained between the vaccine and control groups by Student’s t tests (P value < 0.04). (B) Survival analysis: The Kaplan-Meier curve shows the survival of the following two groups of mice (n = 25): mice that were treated with a vaccine (n = 14) and mice that did not receive a treatment (n = 11). The curve comparison with the log-rank (Mantel-Cox) test revealed statistically significant differences between the vaccine and control groups (P = 0.0002).
In the second strategy, tumorigenesis was done with 4T1 cells, and after tumor induction, mice were divided into three groups. One group received only the cGRP78 vaccine; the second group received the cGRP78 vaccine and doxorubicin; and the third group only received a PBS injection (control). As shown in Figs. 5A and 5B, the mice immunized with the vaccine showed a decrease in tumor volume and an increase in survival. Still, in the second group, the combination of the vaccine and doxorubicin did not show a synergistic effect. Chemotherapy drugs probably suppressed the immune system and reduced the impact of the vaccine. As shown in the Kaplan-Meier plot, 48 days after tumor induction, all mice in the unvaccinated group died, but 80% of the mice in the vaccinated group were alive. The average tumor volumes in the test and control groups were 1373 and 4648 mm3, respectively (P value < 0.0001).
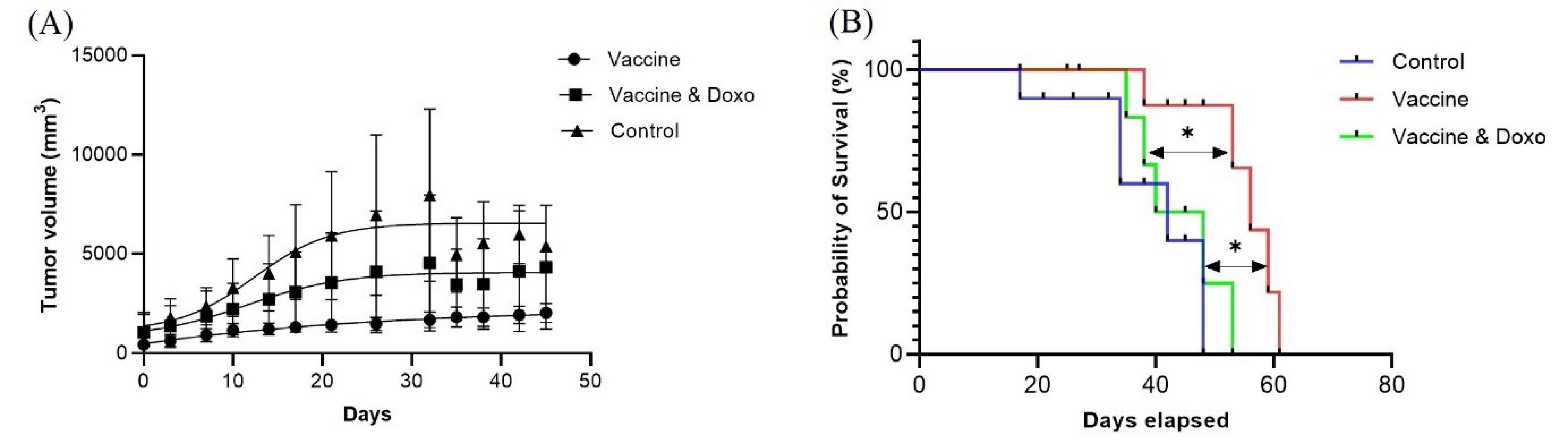
Fig. 5.
(A) Tumor volume change in three groups include vaccine, vaccine – Doxorubicin and control groups: Statistical significance between different groups was obtained by one-way ANOVA using the Tukey post-test, and a significant difference between three groups was indicated (P value < 0.0001). Also, a significant difference was obtained between the vaccine and vaccine-doxorubicin groups by Student’s t tests (P value < 0.0001). The P-value in the comparison of the control group with the vaccine and vaccine-doxorubicin groups was < 0.0001 and < 0.022, respectively. (B) Survival analysis: The Kaplan-Meier curve shows the survival of the following three groups of mice (n = 15): mice treated by vaccine (n = 5), mice treated by vaccine-doxorubicin (n = 5), and mice that did not receive a treatment (n = 5). The curve comparison with the log-rank (Mantel-Cox) test revealed statistically significant differences between vaccine and vaccine-doxorubicin groups (P = 0.018); and between vaccine and control groups (P = 0.012).test revealed statistically significant differences between the vaccine and control groups (P = 0.0002).
Fig. 5.
(A) Tumor volume change in three groups include vaccine, vaccine – Doxorubicin and control groups: Statistical significance between different groups was obtained by one-way ANOVA using the Tukey post-test, and a significant difference between three groups was indicated (P value < 0.0001). Also, a significant difference was obtained between the vaccine and vaccine-doxorubicin groups by Student’s t tests (P value < 0.0001). The P-value in the comparison of the control group with the vaccine and vaccine-doxorubicin groups was < 0.0001 and < 0.022, respectively. (B) Survival analysis: The Kaplan-Meier curve shows the survival of the following three groups of mice (n = 15): mice treated by vaccine (n = 5), mice treated by vaccine-doxorubicin (n = 5), and mice that did not receive a treatment (n = 5). The curve comparison with the log-rank (Mantel-Cox) test revealed statistically significant differences between vaccine and vaccine-doxorubicin groups (P = 0.018); and between vaccine and control groups (P = 0.012).test revealed statistically significant differences between the vaccine and control groups (P = 0.0002).
In vivo tumor treatment and metastasis experiments
To evaluate the capability of the cGRP78 vaccine in the prevention of BC metastasis, cancer cells were injected into the blood of vaccinated and control mice. The lung, liver, and spleen tissues of mice in both groups were examined for cancer nodules. The results showed that after 30 days, the growth of cancer cells was not noticeable in lung and liver tissues (vaccinated and control groups). Still, spleen examination showed that the number of cancer nodules in unvaccinated control mice was significantly higher than in the vaccinated group. To quantify these results, the spleens of both groups of mice were weighed, the results of which are shown in Fig. 6. This showed that the cGRP78 vaccine could significantly reduce the establishment of tumors in the spleen.
Fig. 6.
A comparison of spleen tumor nodules and weight showed a significant difference between the immunized and non-immunized groups (P value < 0.0001).
Fig. 6.
A comparison of spleen tumor nodules and weight showed a significant difference between the immunized and non-immunized groups (P value < 0.0001).
Histopathology experiments
H&E staining of the heart, liver, lung, and kidneys showed no noticeable morphological changes in any of the vaccinated groups (Fig. 7).
Fig. 7.
Pathologic evaluation of the heart, liver, lung, and kidney in control and vaccinated mice: No significant pathologic changes were found between the control (non-immunize) and cGRP78 vaccine groups.
Fig. 7.
Pathologic evaluation of the heart, liver, lung, and kidney in control and vaccinated mice: No significant pathologic changes were found between the control (non-immunize) and cGRP78 vaccine groups.
Discussion
The lack of fully effective therapies and the poor prognosis of breast cancer patients have significantly increased the need to explore molecular targets and develop new therapeutic approaches.16 Non- or low-immunologic cancer cell lines, such as 4T1 cells, can overgrow and escape degradation by the immune system.17
Early treatment protocols for cancerous tumors often include surgery, chemotherapy, and radiotherapy, which often cause the disease to relapse and metastasize. Immunotherapy against cancer is one of the new research fields that generates an active response against tumor surface antigens.18 Immunotherapy against cancer cells is a unique and effective method that activates the immune system against the surface antigens of cancer cells. Because cancer cells can escape the anti-cancer activity of the immune system and rarely expose their antigens to T cells, one of the most important goals of cancer immunotherapy is to make tumor-specific T cells. Another way of using immunotherapy is to use cancer cell surface antigens as a cancer vaccine, which could be a new way to treat tumors. The primary purpose of the vaccine is to induce a specific response against tumors, which can provide long-term immunity to cancer and prevent tumor recurrence. An ideal vaccine can stimulate humoral and cellular immune responses against tumor cells and lead to better treatment of cancer cells.18-20
HSPs are housekeeping proteins that are produced in all cells and organisms but increase in some pathological and physiological conditions, such as heat shock or hypoxia. Because of the high proliferation of cancer cells, they are constantly exposed to a lack of oxygen and nutrients.21 As a result, Hsp70 families, especially those in extracellular and membrane-bound cells, increase their expression in these cells and migrate to the cell surface more than normal cells. APCs are activated against these antigens and can be used in cancer immunotherapy. One member of the sizeable HSP70 family is the 78-kDa protein GRP78, which binds to the hydrophobic residues of unfolded polypeptides in the ER and prevents their accumulation. However, in cancer tissues, because of the increased expression of GRP78 and its placement on the surface of cancer cells, this antigen is a good candidate for cancer immunotherapy.22
Many researchers have developed antibodies against different epitopes of GRP78 and reported the results of using these antibodies. In one study, antibodies against the carboxyl domain of GRP78 in melanoma and prostate cells induced p53 and apoptosis in these cells.23 Using a high-affinity Mab-159 monoclonal antibody (Kd = 1.7 nmol/L) against surface GRP78 in colon cancer and breast carcinoma cells has inhibited proliferation in these cells.24 In a wide range of tumors, such as lung, hepatocellular carcinoma, prostate cancer, and breast cancer, high expression of GRP78 induces resistance to a wide range of pharmaceutical agents.25-29 Inhibition of GRP78 causes tumors to become sensitive to drug therapy.30 As a result, it can be suggested that immunotherapy with cGRP78 could limit the rate of tumor growth and increasing the survivability of mice with breast tumors.
After vaccinating mice with the cGRP78 protein, the spleens of vaccinated and unvaccinated mice were isolated to examine the stimulatory effect of this antigen on immune cells. In this study, an MTT assay was used to measure the suppressive or proliferation effects of the cGRP78 antigen on splenocytes. This antigen notably enhanced the proliferative response of the vaccinated mice's splenocytes more noticeably than the non-vaccinated mice's splenocytes, which shows the cGRP78 vaccine has a stimulation effect on the growth and proliferation of immune cells.
In the next step, a cytokine assay was performed to evaluate the response of immune cells to the cGRP78 antigen. In this test, five cytokines (IL-2, IFN-γ, TGF-β, IL-6, and IL-10) were assessed, with the results shown in Fig. 3.
Previous studies show that IFN-γ levels are associated with an anti-tumor response of the immune system. Macrophages and NK cells (Natural killer cells) are essential in producing the anti-malignant cell response to eliminate tumor cells. NK cells secrete IFN-γ to activate the immune system against tumor cells and induce apoptosis in cancer cells. IFN-γ is also directly related to the activation of the anti-angiogenesis response.31-34 The results showed that IFN-γ levels in cGRP78-immunized mice were significantly higher than in unimmunized mice. As a result, it can be concluded that increased secretion of IFN-γ by immune cells in vaccinated mice causes more apoptosis of cancer cells and slows down the metastasis process in these cells.
Tumor cells use the secretion of mediators such as IL-10 and TGF-β to escape the immune system. These cytokines can suppress essential tools of the immune system against tumors, such as macrophages and Th1. IL-10 and TGF-β suppress cell-mediated immunity by inhibiting the activation and proliferation of macrophages and lymphocytes, which is an essential factor in controlling tumor growth. These cytokines also impede the response of T cells to tumor cells by inducing the development of regulatory T cells. Regulatory T cells are another source for further production of the cytokines IL-10 and TGF-β.35,36 Our study shows that the cGRP78 vaccine reduced TGF-β levels in immunized mice compared to control mice, which prevented cancer cells from suppressing the immune system.
Among the cytokines studied in this study, IL-2 has a regulatory function, activates NK cells, and is the main growth factor for T and B lymphocytes. The research demonstrated that expression of IL-2 was effectively linked to breast cancer progression due to the fact that decreasing the serum concentration of this interleukin is directly related to increasing the relapse rate in breast cancer. This study showed that the vaccine used not only did not reduce IL-2 secretion but also slightly increased it.17,37,38
Administration of the cGRP78 vaccine effectively increases the expression of Th1-specific cytokines, including IL-2 and INFγ, and decreases Th2-specific cytokines such as IL10.17 Therefore, it can be concluded that the cGRP78 vaccine can change the balance of T helper cells towards Th1 production and cause more effective treatment against cancer cells.
Our results showed that the effect of vaccination with this antigen not only reduces the growth of tumor tissue but also increases the lifespan of vaccinated mice. In the strategy of administering the vaccine before tumor inoculation, the results showed that the cGRP78 vaccine fought the initiation of cancer cell growth in immunized mice and delayed this growth. Also, a significant decrease in tumor volume is clear in vaccinated mice. In the second strategy, where the cGRP78 vaccine is administered after tumor inoculation, a reduction in tumor volume can be observed in vaccinated mice. The simultaneous use of doxorubicin with the cGRP78 vaccine showed that the chemotherapy drug could not have a synergistic effect because it weakens the immune system of vaccinated mice and reduces the impact of the vaccine. Also, test results for injecting cancer cells into the blood showed that vaccinated mice were more resistant to cancer cell metastasis and that the immune system of these mice was more effective in preventing the spread of cancer cells.
Conclusion
Our evaluation of the cGRP78 vaccination may open a new approach to the clinical management of patients with breast cancer. This in vivo investigation indicates that cGRP78 could be helpful and a good target as a new vaccine for the treatment of breast cancer and other cancerous tumors.
Research Highlights
What is the current knowledge?
√ GRP78 is a cancer cell-specific biomarker and a beneficial target for cancer treatment.
What is new here?
√ cGRP78 could be helpful as a recombinant vaccine for the treatment of breast cancer.
Acknowledgements
This paper was funded by the Isfahan University of Medical Sciences (Grant No. 198261).
Competing Interests
The authors declare no conflict of interest.
Ethical Statement
In the study, all the steps of working with mice have been done according to the Animal Welfare Guidelines, and the proposal of this research was approved by the Institutional Ethical Committee and Research Advisory Committee of Isfahan University of Medical Sciences in Iran (Ethical code: IR.MUI.RESEARCH.REC.1398.846).
Funding
This paper was funded by the Isfahan University of Medical Sciences (Grant No. 198261).
References
- Aghamollaei H, Ghanei M, Rasaee MJ, Latifi AM, Bakherad H, Fasihi‐Ramandi M, et al. Isolation and characterization of a novel nanobody for detection of GRP78 expressing cancer cells. Biotechnol Appl Biochem 2021. 68: 239-46. 10.1002/bab.1916.
- Farshbaf M, Khosroushahi AY, Mojarad-Jabali S, Zarebkohan A, Valizadeh H, Walker PR. Cell surface GRP78: An emerging imaging marker and therapeutic target for cancer. J Control Release 2020; 328:932-41. doi: 10.1016/j.jconrel.2020.10.055 [Crossref] [ Google Scholar]
- Lee AS. Glucose-regulated proteins in cancer: molecular mechanisms and therapeutic potential. Nat Rev Cancer 2014; 14:263-76. doi: 10.1038/nrc3701 [Crossref] [ Google Scholar]
- Li Z, Li Z. Glucose regulated protein 78: a critical link between tumor microenvironment and cancer hallmarks. Biochim Biophys Acta Rev Cancer 2012; 1826:13-22. doi: 10.1016/j.bbcan.2012.02.001 [Crossref] [ Google Scholar]
- Gifford JB, Hill R. GRP78 influences chemoresistance and prognosis in cancer. Curr Drug Targets 2018; 19:701-8. doi: 10.2174/1389450118666170615100918 [Crossref] [ Google Scholar]
- Ni M, Zhang Y, Lee AS. Beyond the endoplasmic reticulum: atypical GRP78 in cell viability, signalling and therapeutic targeting. Biochem J 2011; 434:181-8. doi: 10.1042/BJ20101569 [Crossref] [ Google Scholar]
- Zhang J, Jiang Y, Jia Z, Li Q, Gong W, Wang L. Association of elevated GRP78 expression with increased lymph node metastasis and poor prognosis in patients with gastric cancer. Clin Exp Metastasis 2006; 23:401-10. doi: 10.1007/s10585-006-9051-9 [Crossref] [ Google Scholar]
- Yao X, Liu H, Zhang X, Zhang L, Li X, Wang C. Cell surface GRP78 accelerated breast cancer cell proliferation and migration by activating STAT3. PloS one 2015; 10:e0125634. doi: 10.1371/journal.pone.0125634 [Crossref] [ Google Scholar]
- Hernandez I, Cohen M. Linking cell-surface GRP78 to cancer: From basic research to clinical value of GRP78 antibodies. Cancer Lett 2022; 524:1-14. doi: 10.1016/j.canlet.2021.10.004 [Crossref] [ Google Scholar]
- Aghamollaei H, Mousavi Gargari SL, Ghanei M, Rasaee MJ, Amani J, Bakherad H. Structure prediction, expression, and antigenicity of c‐terminal of GRP78. Biotechnol Appl Biochem 2017; 64:117-25. doi: 10.1002/bab.1455 [Crossref] [ Google Scholar]
- Parvizpour S, Razmara J, Pourseif MM, Omidi Y. In silico design of a triple-negative breast cancer vaccine by targeting cancer testis antigens. BIBioImpacts: BI 2019; 9:45. doi: 10.15171/bi.2019.06 [Crossref] [ Google Scholar]
- Benedetti R, Dell’Aversana C, Giorgio C, Astorri R, Altucci L. Breast cancer vaccines: new insights. Front Endocrinol 2017; 8:270. doi: 10.3389/fendo.2017.00270 [Crossref] [ Google Scholar]
- Liu J, Fu M, Wang M, Wan D, Wei Y, Wei X. Cancer vaccines as promising immuno-therapeutics: platforms and current progress. J Hematol Oncol 2022; 15:1-26. doi: 10.1186/s13045-022-01247-x [Crossref] [ Google Scholar]
- Hu Z, Ott PA, Wu CJ. Towards personalized, tumour-specific, therapeutic vaccines for cancer. Nat Rev Immunol 2018; 18:168-82. doi: 10.1038/nri.2017.131 [Crossref] [ Google Scholar]
- Ladjemi MZ, Jacot W, Chardès T, Pèlegrin A, Navarro-Teulon I. Anti-HER2 vaccines: new prospects for breast cancer therapy. Cancer Immunol Immunother 2010; 59:1295-312. doi: 10.1007/s00262-010-0869-2 [Crossref] [ Google Scholar]
- Liu L, Wang Y, Miao L, Liu Q, Musetti S, Li J. Combination immunotherapy of MUC1 mRNA nano-vaccine and CTLA-4 blockade effectively inhibits growth of triple negative breast cancer. Mol Ther 2018; 26:45-55. doi: 10.1016/j.ymthe.2017.10.020 [Crossref] [ Google Scholar]
- Jafari S, Froushani SMA, Tokmachi A. Combined extract of heated 4T1 and a heat-killed preparation of Lactobacillus casei in a mouse model of breast cancer. Iran J Med Sci 2017; 42:457. [ Google Scholar]
- Gheybi E, Salmanian AH, Fooladi AAI, Salimian J, Hosseini HM, Halabian R. Immunogenicity of chimeric MUC1-HER2 vaccine against breast cancer in mice. Iran J Basic Med Sci 2018; 21:26. doi: 10.22038/IJBMS.2017.25686.6335 [Crossref] [ Google Scholar]
- Soliman H. Developing an effective breast cancer vaccine. Cancer Control 2010; 17:183-90. doi: 10.1177/107327481001700307 [Crossref] [ Google Scholar]
- Lakshminarayanan V, Thompson P, Wolfert MA, Buskas T, Bradley JM, Pathangey LB. Immune recognition of tumor-associated mucin MUC1 is achieved by a fully synthetic aberrantly glycosylated MUC1 tripartite vaccine. Proc Natl Acad Sci USA 2012; 109:261-6. doi: 10.1073/pnas.1115166109 [Crossref] [ Google Scholar]
- Mahmood K, Jadoon S, Mahmood Q, Irshad M, Hussain J. Synergistic effects of toxic elements on heat shock proteins. Biomed Res Int 2014; 2014:564136. doi: 10.1155/2014/564136 [Crossref] [ Google Scholar]
- Ciocca DR, Cayado-Gutierrez N, Maccioni M, Cuello-Carrion FD. Heat shock proteins (HSPs) based anti-cancer vaccines. Curr Mol Med 2012; 12:1183-97. doi: 10.2174/156652412803306684 [Crossref] [ Google Scholar]
- Misra UK, Mowery Y, Kaczowka S, Pizzo SV. Ligation of cancer cell surface GRP78 with antibodies directed against its COOH-terminal domain up-regulates p53 activity and promotes apoptosis. Mol Cancer Ther 2009; 8:1350-62. doi: 10.1158/1535-7163.MCT-08-0990 [Crossref] [ Google Scholar]
- Liu R, Li X, Gao W, Zhou Y, Wey S, Mitra SK. Monoclonal Antibody against Cell Surface GRP78 as a Novel Agent in Suppressing PI3K/AKT Signaling, Tumor Growth, and MetastasisGRP78 Antibody Inhibits PI3K and Tumor Growth. Clin Cancer Res 2013; 19:6802-11. doi: 10.1158/1078-0432.CCR-13-1106 [Crossref] [ Google Scholar]
- Uramoto H, Sugio K, Oyama T, Nakata S, Ono K, Yoshimastu T. Expression of endoplasmic reticulum molecular chaperone Grp78 in human lung cancer and its clinical significance. Lung Cancer 2005; 49:55-62. doi: 10.1016/j.lungcan.2004.12.011 [Crossref] [ Google Scholar]
- Shuda M, Kondoh N, Imazeki N, Tanaka K, Okada T, Mori K. Activation of the ATF6, XBP1 and grp78 genes in human hepatocellular carcinoma: a possible involvement of the ER stress pathway in hepatocarcinogenesis. J Hepatol 2003; 38:605-14. doi: 10.1016/S0168-8278(03)00029-1 [Crossref] [ Google Scholar]
- Daneshmand S, Quek ML, Lin E, Lee C, Cote RJ, Hawes D. Glucose-regulated protein GRP78 is up-regulated in prostate cancer and correlates with recurrence and survival. Hum Pathol 2007; 38:1547-52. doi: 10.1016/j.humpath.2007.03.014 [Crossref] [ Google Scholar]
- Zhou H, Zhang Y, Fu Y, Chan L, Lee AS. Novel mechanism of anti-apoptotic function of 78-kDa glucose-regulated protein (GRP78): endocrine resistance factor in breast cancer, through release of B-cell lymphoma 2 (BCL-2) from BCL-2-interacting killer (BIK). J Biol Chem 2011; 286:25687-96. doi: 10.1074/jbc.M110.212944 [Crossref] [ Google Scholar]
- Scriven P, Coulson S, Haines R, Balasubramanian S, Cross S, Wyld L. Activation and clinical significance of the unfolded protein response in breast cancer. Br J Cancer 2009; 101:1692-8. doi: 10.1038/sj.bjc.6605365 [Crossref] [ Google Scholar]
- Cook KL, Clarke R. Role of GRP78 in promoting therapeutic-resistant breast cancer. Future Med Chem 2015; 7:1529-34. doi: 10.4155/fmc.15.80 [Crossref] [ Google Scholar]
- Josephs DH, Bax HJ, Karagiannis SN. Tumour-associated macrophage polarisation and re-education with immunotherapy. Front Biosci (Elite Ed) 2015; 7:293-308. doi: 10.2741/E735 [Crossref] [ Google Scholar]
- Khatibi AS, Roodbari NH, Majidzade-A K, Yaghmaei P, Farahmand L. In vivo tumor-suppressing and anti-angiogenic activities of a recombinant anti-CD3ε nanobody in breast cancer mice model. Immunotherapy 2019; 11:1555-67. doi: 10.2217/imt-2019-0068 [Crossref] [ Google Scholar]
- Pahl J, Cerwenka A. Tricking the balance: NK cells in anti-cancer immunity. Immunobiology 2017; 222:11-20. doi: 10.1016/j.imbio.2015.07.012 [Crossref] [ Google Scholar]
- Razazan A, Behravan J, Arab A, Barati N, Arabi L, Gholizadeh Z. Conjugated nanoliposome with the HER2/neu-derived peptide GP2 as an effective vaccine against breast cancer in mice xenograft model. PloS One 2017; 12:e0185099. doi: 10.1371/journal.pone.0185099 [Crossref] [ Google Scholar]
- Austenaa LM, Carlsen H, Ertesvag A, Alexander G, Blomhoff HK, Blomhoff R. Vitamin A status significantly alters nuclear factor‐κ B activity assessed by in vivo imaging. The FASEB journal 2004; 18:1255-7. doi: 10.1096/fj.03-1098fje [Crossref] [ Google Scholar]
- Wobke T, Sorg B, Steinhilber D. Vitamin D in inflammatory diseases. Front Physiol 2014; 5:244. doi: 10.3389/fphys.2014.00244 [Crossref] [ Google Scholar]
- Burns L, Weisdorf D, DeFor T, Vesole D, Repka T, Blazar B. IL-2-based immunotherapy after autologous transplantation for lymphoma and breast cancer induces immune activation and cytokine release: a phase I/II trial. Bone Marrow Transplant 2003; 32:177-86. doi: 10.1038/sj.bmt.1704086 [Crossref] [ Google Scholar]
- Knutson KL, Dang Y, Lu H, Lukas J, Almand B, Gad E. IL-2 immunotoxin therapy modulates tumor-associated regulatory T cells and leads to lasting immune-mediated rejection of breast cancers in neu-transgenic mice. J Immunol 2006; 177:84-91. doi: 10.4049/jimmunol.177.1.84 [Crossref] [ Google Scholar]